The Estuarine Food Chain, like a vibrant tapestry woven by the sea, is a bustling community where life thrives at the meeting point of rivers and oceans. These unique environments, often cradled by the embrace of land, are nurseries of abundance, brimming with life that supports both aquatic and terrestrial creatures. From the tiniest phytoplankton to the apex predators, a delicate balance exists, where every organism plays a vital role in the symphony of life that defines the estuary.
Estuaries, acting as nature’s grand transition zones, are where freshwater rivers meet the salty embrace of the sea, creating brackish waters that nurture an array of life. These areas, distributed globally, are cradles of biodiversity, providing essential habitats and sustaining ecosystems. Within these unique ecosystems, primary producers like phytoplankton, seagrasses, mangroves, and salt marshes form the base, fueling a chain that supports an intricate web of life.
From herbivores munching on the vegetation to carnivores stalking their prey, the estuarine food chain exemplifies nature’s ability to support and regulate life.
Introduction to Estuarine Ecosystems
Estuaries are among the most biologically productive ecosystems on Earth, serving as critical habitats for a wide variety of plant and animal life. These dynamic environments are characterized by a unique blend of freshwater and saltwater, creating a gradient of salinity and other environmental factors that shape the ecological communities found within them. Understanding the characteristics, significance, and global distribution of estuaries is crucial for effective conservation and management of these valuable ecosystems.
Definition and Key Characteristics
An estuary is a partially enclosed coastal body of water where freshwater from rivers and streams mixes with saltwater from the ocean. This mixing creates a brackish environment, with salinity levels varying both spatially and temporally. Estuaries are characterized by several key features that distinguish them from other coastal habitats.
- Salinity Gradients: The mixing of freshwater and saltwater results in a salinity gradient, with lower salinity levels near the river’s mouth and increasing salinity towards the ocean. This gradient is a primary factor influencing the distribution of estuarine organisms.
- Tidal Influence: Estuaries are strongly influenced by tides, which affect water levels, currents, and the exchange of water with the ocean. Tidal fluctuations also influence the distribution of intertidal habitats, such as mudflats and salt marshes.
- Sedimentation: Estuaries are often characterized by high rates of sedimentation, as rivers transport sediment and nutrients into the estuary. This sediment accumulation can create intertidal habitats and influence water clarity.
- Nutrient Richness: Estuaries are generally nutrient-rich environments due to the influx of nutrients from both freshwater and saltwater sources. These nutrients support high levels of primary productivity, forming the base of the estuarine food web.
Significance as Transitional Zones
Estuaries serve as crucial transitional zones between terrestrial, freshwater, and marine ecosystems, providing a variety of ecological functions and benefits. These functions contribute significantly to the overall health and productivity of coastal regions.
- Habitat for Diverse Species: Estuaries provide habitat for a wide range of species, including fish, shellfish, birds, and marine mammals. Many species use estuaries as nurseries, feeding grounds, or migratory stopover points. For example, the Chesapeake Bay in the United States is a vital nursery for commercially important fish species like striped bass.
- Water Filtration and Purification: Estuarine ecosystems can filter pollutants and excess nutrients from both freshwater and saltwater sources. Salt marshes and other estuarine habitats can absorb pollutants and remove excess nutrients, improving water quality.
- Coastal Protection: Estuarine habitats, such as mangroves and salt marshes, can help protect coastlines from erosion and storm surges. The vegetation in these habitats can absorb wave energy and stabilize sediments, reducing the impact of coastal storms. For instance, mangroves in Florida have been shown to reduce the impact of hurricanes on coastal communities.
- Economic Value: Estuaries support significant economic activities, including fisheries, tourism, and recreation. Estuarine fisheries are often highly productive, providing a valuable food source and generating income for coastal communities. The oyster industry in the Gulf of Mexico is a prime example of the economic importance of estuaries.
Geographical Distribution of Estuaries
Estuaries are found worldwide, along coastlines where rivers meet the ocean. Their distribution is influenced by factors such as climate, topography, and river flow.
- Global Distribution: Estuaries occur on every continent except Antarctica. They are particularly common along coastlines with extensive river systems and shallow coastal waters. Examples include the Amazon River estuary in South America, the Ganges-Brahmaputra Delta in Asia, and the Mississippi River Delta in North America.
- Types of Estuaries: The classification of estuaries is based on their formation, salinity, and mixing patterns. These include:
- Drowned River Valleys (Coastal Plain Estuaries): Formed when rising sea levels flood river valleys. The Chesapeake Bay is a prime example.
- Bar-Built Estuaries: Formed when sandbars or barrier islands partially enclose a coastal area. The Outer Banks of North Carolina feature numerous bar-built estuaries.
- Tectonic Estuaries: Formed by the sinking of land due to tectonic activity. San Francisco Bay is an example.
- Fjords: Deep, glacially carved valleys that have been flooded by the sea. Found in Norway, New Zealand, and other regions with glacial history.
- Influence of Climate: The characteristics of estuaries vary depending on the climate. In tropical regions, estuaries may be dominated by mangrove forests, while in temperate regions, salt marshes are more common. In colder regions, estuaries may be ice-covered for part of the year, impacting the ecosystem processes.
Primary Producers in the Estuarine Food Chain
Estuaries, characterized by their dynamic interplay of freshwater and saltwater, are incredibly productive ecosystems. This productivity is largely driven by primary producers, organisms that convert sunlight into energy through photosynthesis. These producers form the base of the estuarine food web, supporting a diverse array of life. Their abundance and types vary depending on factors like salinity, light penetration, and substrate availability.
Major Primary Producers in Estuaries
The primary producers in estuaries encompass a range of organisms, from microscopic phytoplankton to large, rooted plants. Understanding the contribution of each type is essential to appreciating the overall functioning of the estuarine ecosystem.
- Phytoplankton: These microscopic, free-floating algae are the dominant primary producers in many estuaries. They are highly efficient at capturing sunlight and converting it into organic matter. Diatoms, dinoflagellates, and cyanobacteria are common examples. Their rapid growth rates contribute significantly to the overall primary productivity of the estuary.
- Macroalgae: Also known as seaweeds, macroalgae such as green algae (e.g., Ulva), brown algae (e.g., Fucus), and red algae (e.g., Gracilaria) can be significant primary producers, particularly in areas with hard substrates or where light penetration is sufficient. They provide habitat and food for various estuarine organisms.
- Estuarine Vegetation: This category includes rooted, vascular plants that are adapted to the estuarine environment. These plants are critical in stabilizing sediments, providing habitat, and contributing organic matter to the food web. Examples include salt marsh grasses, mangroves, and seagrasses.
Role of Phytoplankton in the Estuarine Food Web
Phytoplankton play a pivotal role in the estuarine food web, serving as the primary energy source for a multitude of consumers. Their abundance and composition influence the entire ecosystem.
Phytoplankton are consumed directly by zooplankton, tiny animals that graze on these microscopic algae. Zooplankton, in turn, are preyed upon by small fish and invertebrates, which are then consumed by larger predators. This transfer of energy from phytoplankton to higher trophic levels demonstrates the fundamental role of phytoplankton in supporting the estuarine food web. Furthermore, phytoplankton contribute to the oxygen levels in the water through photosynthesis.
Comparison of Estuarine Vegetation Types
Different types of estuarine vegetation have unique adaptations and ecological roles. A comparison of salt marshes, mangroves, and seagrass beds reveals their diverse contributions to the estuarine ecosystem.
Feature | Salt Marshes | Mangroves | Seagrass Beds |
---|---|---|---|
Dominant Plant Type | Grasses and herbaceous plants (e.g., Spartina alterniflora) | Trees and shrubs (e.g., Rhizophora mangle) | Submerged flowering plants (e.g., Zostera marina) |
Typical Location | Temperate and high-latitude regions; intertidal zones with fine sediments | Tropical and subtropical regions; intertidal zones with muddy sediments | Shallow, subtidal and intertidal areas; sandy or muddy substrates |
Adaptations to Salinity | Salt tolerance mechanisms (e.g., salt glands, salt exclusion) | Salt exclusion and excretion mechanisms | Salt tolerance; specialized cells for ion regulation |
Ecological Roles |
|
|
|
Primary Consumers: The Herbivores
Herbivores are a critical link in the estuarine food web, transferring energy from primary producers to higher trophic levels. Their feeding activities profoundly influence the structure and function of the estuarine ecosystem, driving nutrient cycling and shaping the distribution of plant communities.
Role of Herbivores in the Estuarine Ecosystem
Herbivores consume primary producers, such as phytoplankton, macroalgae, and submerged aquatic vegetation (SAV). This grazing activity regulates the abundance and composition of these primary producers, preventing them from overgrowing and potentially shading out other organisms. Herbivores also contribute to nutrient cycling by consuming plant material and excreting waste products, releasing nutrients back into the water column or sediment. These released nutrients are then available for uptake by primary producers, thus driving the cycle.
Additionally, the grazing activities of herbivores can influence the physical structure of the estuarine habitat, for example, by preventing the build-up of detritus or promoting the growth of specific plant species.
Common Estuarine Herbivores
A diverse array of animals acts as herbivores in estuarine ecosystems. These include:
- Zooplankton: These microscopic animals, such as copepods and cladocerans, are a vital food source for many estuarine animals. They feed on phytoplankton, controlling algal blooms and transferring energy to larger organisms.
- Benthic Invertebrates: Several invertebrate species graze on algae and SAV.
- Gastropods (Snails): Snails, such as periwinkles ( Littorina spp.), are common grazers on macroalgae and biofilms on submerged surfaces. They use their radula (a toothed, tongue-like structure) to scrape and consume plant material.
- Crustaceans: Crustaceans like amphipods and isopods graze on detritus, macroalgae, and the epiphytes growing on SAV.
- Fish: Some fish species are primarily herbivorous or omnivorous, feeding on plants and algae. Examples include:
- Mullet (Mugil spp.): Mullet are known for their ability to graze on algae and detritus, playing a role in the nutrient cycling within the ecosystem.
- Some Surgeonfish: In tropical estuaries, some surgeonfish species graze on algae, contributing to the regulation of algal growth.
- Marine Mammals:
- Manatees (Trichechus manatus): In certain estuaries, manatees graze on SAV, significantly influencing the distribution and abundance of these plants. Their feeding habits can create clearings in seagrass beds, which can affect the habitat for other species.
Impact of Herbivore Grazing on Primary Producers
Herbivore grazing has significant impacts on primary producers. The intensity of grazing can vary based on herbivore abundance, species, and environmental conditions.
- Regulation of Algal Blooms: Zooplankton grazing on phytoplankton can help to control the size and duration of algal blooms. Intense grazing can prevent excessive phytoplankton growth, which can lead to hypoxia (low oxygen conditions) and harm other organisms.
- Control of Macroalgae: Grazing by snails, crabs, and fish on macroalgae can limit their growth and prevent them from outcompeting SAV. In estuaries where macroalgae growth is unchecked, it can smother other organisms and alter the habitat structure.
- Influence on SAV: Herbivores like manatees and some fish species can directly graze on SAV, influencing the structure and density of seagrass beds. Intense grazing can create patches and openings in seagrass beds, altering the habitat for other organisms and affecting sediment stability.
- Nutrient Cycling: By consuming primary producers and excreting waste, herbivores contribute to nutrient cycling. Their waste products contain nutrients that are then available for uptake by primary producers, supporting the growth of the plants and maintaining the balance of the ecosystem.
Secondary Consumers: The Carnivores
Carnivores occupy a critical position in the estuarine food chain, representing a trophic level that feeds primarily on other animals. Their presence significantly influences the structure and function of the ecosystem, controlling populations of herbivores and other carnivores, and contributing to the overall energy flow. They act as regulators, shaping the community composition and influencing the dynamics of nutrient cycling within the estuary.
Role of Carnivores in the Estuarine Food Chain
Carnivores play a vital role in the estuarine food web by consuming other animals, thus transferring energy from lower to higher trophic levels. They exert top-down control, influencing the abundance and distribution of their prey. Their predatory activities help regulate populations of herbivores, preventing overgrazing of primary producers. They also impact the structure of the food web by competing with each other for resources.
For example, larger predatory fish might consume smaller fish, which in turn have been feeding on invertebrates.
Types of Carnivores in Estuaries
Estuaries support a diverse array of carnivores, each adapted to exploit specific prey and habitats. These carnivores can be broadly categorized into fish, birds, and mammals, among others.
Discover how best no till food plot has transformed methods in this topic.
- Fish: Estuarine fish represent a significant component of the carnivore community. They exhibit a wide range of feeding strategies, from ambush predators to active hunters. Examples include:
- Largemouth Bass (Micropterus salmoides): A common freshwater fish that can inhabit brackish waters in estuaries. They are ambush predators, consuming fish, crustaceans, and other invertebrates.
- Striped Bass (Morone saxatilis): A migratory species that spends part of its life cycle in estuaries. They are opportunistic predators, feeding on fish, crustaceans, and other invertebrates.
- Bluefish (Pomatomus saltatrix): A highly migratory and voracious predator that enters estuaries to feed. They feed on fish, crustaceans, and squid.
- Birds: Various bird species utilize estuaries as feeding grounds, preying on fish, crustaceans, and other invertebrates. They often exhibit specialized adaptations for capturing prey. Examples include:
- Herons and Egrets (Family Ardeidae): These wading birds use their sharp beaks to spear fish and other aquatic animals.
- Ospreys (Pandion haliaetus): Specialized fish-eating raptors with strong talons for capturing their prey.
- Kingfishers (Family Alcedinidae): Dive from perches to capture fish and crustaceans.
- Mammals: Marine mammals, such as seals and dolphins, also utilize estuaries for feeding. Their presence adds another layer of complexity to the food web. Examples include:
- Harbor Seals (Phoca vitulina): These seals consume fish and invertebrates.
- Bottlenose Dolphins (Tursiops truncatus): Found in some estuaries, feeding on fish.
Feeding Strategies of Estuarine Carnivores
Estuarine carnivores employ a variety of feeding strategies to obtain their prey, reflecting the diversity of available food resources and their specific adaptations. These strategies range from active hunting to ambush predation.
- Active Hunting: Some carnivores actively pursue their prey. Fish like bluefish and striped bass use their speed and agility to catch smaller fish. Birds such as ospreys actively hunt fish by diving into the water.
- Ambush Predation: Other carnivores employ ambush tactics. Largemouth bass often hide among vegetation, waiting to ambush passing prey.
- Scavenging: Some carnivores may also scavenge on dead organisms. Crabs and some fish will feed on dead fish or other carcasses.
- Specialized Feeding: Some carnivores have specialized feeding adaptations. Ospreys have rough scales on their feet to grip fish, while herons have long necks and beaks for spearing prey.
Tertiary Consumers and Apex Predators
The pinnacle of an estuarine food web is occupied by tertiary consumers and apex predators. These organisms play a crucial role in regulating the structure and function of the entire ecosystem. Their presence or absence can have cascading effects on the populations of lower trophic levels, influencing everything from the abundance of primary producers to the diversity of invertebrate communities.
Role of Top Predators in Regulating Estuarine Food Webs
Top predators exert top-down control within estuarine ecosystems. They influence the abundance and behavior of their prey, which, in turn, impacts the populations of organisms at lower trophic levels. This trophic cascade effect is a fundamental concept in ecology, highlighting the interconnectedness of species within a food web. For instance, the presence of a large predator, like a shark, can reduce the number of mid-level predators, such as larger fish, which then allows populations of smaller fish and crustaceans to increase.
This can ultimately affect the grazing pressure on primary producers, such as seagrass, influencing the overall health and productivity of the estuary. The strength of these top-down effects varies depending on the specific species involved, the complexity of the food web, and environmental conditions.
Examples of Apex Predators in Estuaries
Estuaries are home to a variety of apex predators, each playing a specific role in the ecosystem. These predators often exhibit high mobility and broad diets, allowing them to exploit resources across different habitats within the estuary.Examples include:
- Sharks: Several shark species, such as bull sharks ( Carcharhinus leucas) and tiger sharks ( Galeocerdo cuvier), are common apex predators in estuaries, particularly in warmer regions. They prey on a wide range of organisms, including fish, marine mammals, and even other sharks.
- Large Fish: Species like the Atlantic tarpon ( Megalops atlanticus), and the alligator gar ( Atractosteus spatula) function as apex predators, feeding on smaller fish and invertebrates. These fish often require specific habitat features, such as deep channels or submerged vegetation, to thrive.
- Marine Mammals: Some estuarine environments support populations of marine mammals, such as bottlenose dolphins ( Tursiops truncatus). These intelligent predators feed on fish and other marine life, contributing significantly to the estuarine food web dynamics. They have complex social structures and are sensitive to human disturbances.
- Birds: Various bird species, including ospreys ( Pandion haliaetus) and brown pelicans ( Pelecanus occidentalis), also function as apex predators in estuaries. They feed on fish, crustaceans, and other aquatic organisms, contributing to the top-down control within the ecosystem. These birds often rely on healthy fish populations and undisturbed nesting sites.
Threats Facing Apex Predators in Estuaries
Apex predators in estuaries face a multitude of threats, many of which are directly or indirectly linked to human activities. These threats can lead to population declines, disrupt ecosystem balance, and reduce the overall resilience of the estuarine environment.Some significant threats include:
- Habitat Loss and Degradation: The destruction and alteration of estuarine habitats, such as wetlands, mangroves, and seagrass beds, can reduce the availability of prey, spawning grounds, and shelter for apex predators. Coastal development, pollution, and climate change all contribute to habitat loss.
- Overfishing: Overfishing of prey species can lead to food scarcity for apex predators. Additionally, direct fishing of apex predators, such as sharks, can decimate their populations, disrupting the food web and leading to ecological imbalances. Bycatch, where non-target species are caught in fishing gear, is a significant threat to many apex predators.
- Pollution: Estuaries are often exposed to various forms of pollution, including chemical contaminants, plastic debris, and nutrient runoff. These pollutants can accumulate in apex predators through biomagnification, leading to health problems, reproductive impairment, and increased mortality.
- Climate Change: Climate change can affect apex predators in several ways. Rising sea levels can inundate habitats, altering water salinity and temperature, which may affect prey availability and predator distributions. Changes in ocean currents and weather patterns can disrupt migration routes and feeding patterns.
- Human Disturbance: Increased human activity, such as boating, tourism, and recreational fishing, can disturb apex predators, especially during breeding and feeding periods. Noise pollution and physical disturbance can stress these animals and disrupt their natural behaviors.
Detritus and Decomposers
The estuarine food web is significantly shaped by the detritus pathway, a crucial energy transfer mechanism. Detritus, composed of dead organic matter, fuels a substantial portion of the estuarine ecosystem. Decomposers break down this organic material, recycling nutrients and providing a vital food source for many organisms. This process underscores the interconnectedness of life within the estuary, highlighting the essential role of detritus in supporting overall ecosystem health and productivity.
Importance of Detritus in the Estuarine Food Chain
Detritus is the cornerstone of energy flow in many estuarine ecosystems. It provides a continuous and abundant food source, especially in environments where primary production by phytoplankton and macrophytes may fluctuate seasonally or geographically.The significance of detritus in the estuarine food chain can be summarized as follows:
- Primary Energy Source: Detritus serves as the primary energy source for a significant proportion of estuarine organisms, including many invertebrates and smaller fish. It provides a stable food base, unlike the potentially variable availability of living primary producers.
- Nutrient Recycling: Decomposers break down detritus, releasing essential nutrients like nitrogen and phosphorus back into the water column and sediments. These nutrients are then available for uptake by primary producers, fueling further growth and sustaining the cycle.
- Habitat Creation: Detritus, particularly in the form of decaying plant matter like mangrove leaves or seagrass, can create complex habitats. These habitats offer shelter and foraging opportunities for various organisms, contributing to biodiversity.
- Food Web Support: Detritus supports a complex food web, indirectly feeding larger consumers such as fish and birds. Many invertebrates that consume detritus are, in turn, preyed upon by higher trophic levels.
Main Decomposers in Estuaries
The breakdown of detritus is carried out by a diverse community of decomposers, including bacteria, fungi, and other microorganisms. These organisms play a critical role in breaking down complex organic molecules into simpler substances.The primary decomposers in estuaries are:
- Bacteria: Bacteria are the most abundant decomposers in estuaries. They are highly efficient at breaking down a wide range of organic materials, including cellulose, lignin, and proteins. Different bacterial species specialize in breaking down specific components of detritus. For example, sulfate-reducing bacteria play a crucial role in anaerobic decomposition, producing hydrogen sulfide as a byproduct.
- Fungi: Fungi, particularly filamentous fungi and yeasts, are also important decomposers. They are particularly effective at breaking down complex plant materials like cellulose and lignin, which are abundant in estuarine detritus. Fungi often colonize dead plant matter, creating a network of hyphae that facilitates decomposition.
- Other Microorganisms: Protozoa, micro-invertebrates (e.g., nematodes, copepods), and other small organisms also contribute to the decomposition process. They feed on bacteria and fungi, further breaking down organic matter and releasing nutrients.
Diagram of the Detritus Pathway in an Estuarine Food Web
The detritus pathway illustrates the flow of energy and nutrients from dead organic matter through the estuarine food web. A simplified diagram could depict this pathway as follows:
Diagram Description:
The diagram depicts a cyclical flow of energy and nutrients within an estuarine ecosystem. The central component is detritus, which originates from various sources, including dead plants (e.g., mangrove leaves, seagrass), dead animals, and waste products. Arrows indicate the direction of energy and nutrient flow.
Components of the Diagram:
- Primary Producers: Represented by plants (e.g., mangroves, seagrass) and phytoplankton. They contribute to detritus when they die or shed parts.
- Detritus: This is the central hub, composed of dead organic matter. It is acted upon by decomposers.
- Decomposers: These include bacteria and fungi, which break down detritus, releasing nutrients.
- Detritivores: Organisms that directly consume detritus. Examples include various invertebrates (e.g., worms, crabs, amphipods).
- Consumers: These organisms feed on detritivores, connecting the detritus pathway to higher trophic levels (e.g., fish, birds).
- Nutrients: Released by decomposers and absorbed by primary producers, closing the cycle.
Pathway Illustration:
- Primary producers (plants and phytoplankton) die or shed parts, contributing to detritus.
- Detritus is broken down by decomposers (bacteria and fungi).
- Decomposers release nutrients back into the water, which are then utilized by primary producers, restarting the cycle.
- Detritivores consume detritus, transferring energy to higher trophic levels.
- Consumers (fish, birds) feed on detritivores and other consumers, connecting the detritus pathway to the rest of the food web.
Energy Flow and Trophic Levels
The flow of energy is a fundamental process that governs the structure and function of estuarine ecosystems. It dictates who eats whom, how much energy is available at each level, and ultimately, the productivity and stability of the entire food web. Understanding energy flow is crucial for comprehending the complex interactions within these dynamic environments.
Energy Transfer Mechanisms
Energy transfer in an estuary follows the laws of thermodynamics, particularly the second law, which states that with each transfer, some energy is lost as heat. This unidirectional flow begins with primary producers, capturing solar energy, and moves up through the various trophic levels as organisms consume each other.
Trophic Levels in an Estuary
Estuaries, like all ecosystems, are organized into trophic levels, representing the feeding relationships within the community. Each level receives energy from the level below, with a significant portion of energy lost at each transfer due to metabolic processes and inefficiencies.
- Primary Producers: These organisms, primarily phytoplankton, seaweeds, and submerged aquatic vegetation (SAV), capture solar energy through photosynthesis. They form the base of the food web, converting light energy into chemical energy in the form of organic molecules. The efficiency of this conversion is often relatively low, with only a small percentage of the available sunlight being converted into usable energy.
- Phytoplankton (e.g., diatoms, dinoflagellates): Microscopic algae that drift in the water column.
- Seaweeds (e.g., kelp,
-Ulva*): Larger, multicellular algae that attach to the substrate. - SAV (e.g., eelgrass,
-Zostera marina*): Rooted plants that grow submerged in the estuarine environment.
- Primary Consumers (Herbivores): These organisms feed directly on primary producers, obtaining energy from the organic matter produced through photosynthesis. They represent the second trophic level.
- Zooplankton (e.g., copepods, krill): Microscopic animals that graze on phytoplankton.
- Herbivorous invertebrates (e.g., snails, amphipods): Invertebrates that feed on seaweeds or SAV.
- Herbivorous fish (e.g., mullet): Fish that graze on algae or SAV.
- Secondary Consumers (Carnivores): These organisms consume primary consumers, obtaining energy from the herbivores. They occupy the third trophic level.
- Carnivorous invertebrates (e.g., crabs, worms): Invertebrates that prey on zooplankton or other small invertebrates.
- Carnivorous fish (e.g., small fish, juvenile stages of larger predators): Fish that feed on zooplankton, small invertebrates, or other small fish.
- Tertiary Consumers and Apex Predators: These organisms consume secondary consumers, occupying higher trophic levels. Apex predators are at the top of the food web and are not typically preyed upon by other organisms within the estuary.
- Larger carnivorous fish (e.g., striped bass, bluefish): Fish that prey on smaller fish.
- Marine mammals (e.g., seals, dolphins): Mammals that feed on fish.
- Birds (e.g., herons, osprey): Birds that consume fish and other marine life.
- Detritivores and Decomposers: Detritus, consisting of dead organic matter, is consumed by detritivores, such as certain worms and crustaceans. Decomposers, primarily bacteria and fungi, break down dead organic matter, returning nutrients to the ecosystem. These organisms play a crucial role in recycling nutrients and maintaining the flow of energy within the estuarine ecosystem.
- Bacteria: Microscopic organisms that break down organic matter.
- Fungi: Multicellular organisms that decompose organic material.
- Detritivorous invertebrates (e.g., worms, crabs): Invertebrates that feed on detritus.
The transfer of energy between trophic levels is not perfectly efficient. Typically, only about 10% of the energy at one trophic level is transferred to the next. The remaining energy is lost as heat due to metabolic processes, or is unavailable because it is in the form of undigested material or waste. This concept is often illustrated by the ecological pyramid.
Factors Influencing the Estuarine Food Chain
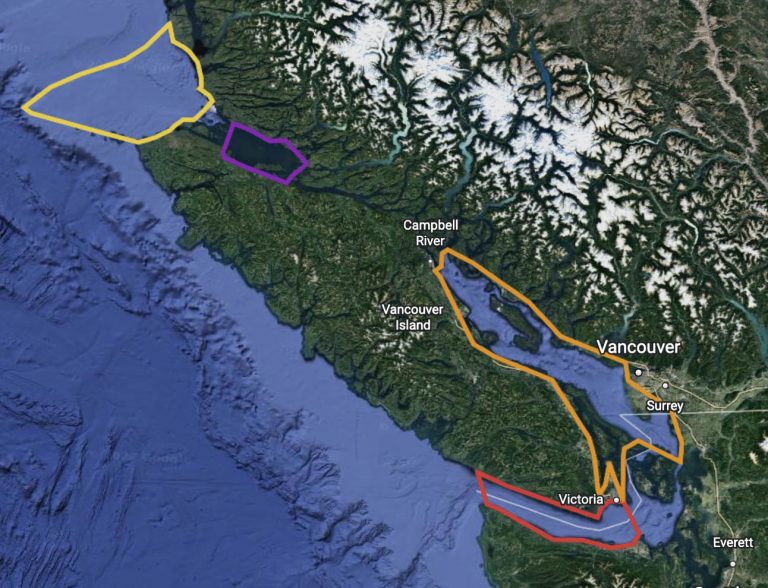
Estuarine food chains are dynamic and intricately linked to a variety of environmental factors. These factors, both natural and anthropogenic, can significantly impact the structure, function, and overall health of these critical ecosystems. Understanding these influences is crucial for effective conservation and management strategies.
Impact of Salinity on Estuarine Food Webs
Salinity, the salt content of water, is a defining characteristic of estuaries, fluctuating widely due to tidal cycles, freshwater input from rivers, and evaporation. This variability profoundly influences the distribution and abundance of estuarine organisms, thereby shaping the food web.Salinity acts as a physiological stressor, influencing the ability of organisms to osmoregulate (maintain internal salt and water balance). Organisms have different salinity tolerances, leading to distinct zonation patterns within the estuary.
For instance:
- Euryhaline species: These organisms, such as the blue crab (
-Callinectes sapidus* ) and many species of fish, can tolerate a wide range of salinities and are often found throughout the estuary. - Stenohaline species: These organisms, like certain marine invertebrates, have narrow salinity tolerances and are typically restricted to either the higher-salinity mouth of the estuary or the lower-salinity freshwater inflow.
The salinity gradient creates distinct habitats, supporting different communities. Areas with lower salinity near river mouths may be dominated by freshwater species and estuarine-tolerant species, while the higher-salinity mouth of the estuary hosts a more diverse array of marine organisms. Changes in salinity, such as those caused by heavy rainfall, droughts, or human interventions like dam construction, can disrupt these habitats and food web structures.
For example, prolonged periods of low salinity can reduce the populations of salt-tolerant species, impacting the food supply for their predators. Conversely, increased salinity can allow marine predators to move further into the estuary, potentially impacting the native estuarine species.
Influence of Nutrient Availability on Estuarine Productivity
Nutrient availability, particularly of nitrogen and phosphorus, plays a crucial role in regulating primary productivity in estuaries. These nutrients are essential for the growth of phytoplankton, the base of the estuarine food web.Nutrients enter estuaries from various sources:
- River runoff: Carries nutrients from agricultural lands, urban areas, and natural watersheds.
- Atmospheric deposition: Nutrients from air pollution can enter the estuary.
- Upwelling: In some estuaries, nutrient-rich deep water can be brought to the surface.
- Sediment release: Nutrients stored in bottom sediments can be released back into the water column.
Increased nutrient levels can lead to eutrophication, a process characterized by excessive algal growth (algal blooms). While initially increasing primary productivity, excessive algal blooms can have negative consequences:
- Oxygen depletion: As algae die and decompose, bacteria consume oxygen, leading to hypoxia (low oxygen levels) or anoxia (no oxygen), which can kill fish and other aquatic organisms.
- Harmful algal blooms (HABs): Some algal species produce toxins that can harm or kill marine life and pose risks to human health through shellfish consumption.
- Reduced light penetration: Dense algal blooms can block sunlight, reducing the growth of submerged aquatic vegetation (SAV), which provides habitat and food for many estuarine organisms.
Nutrient management strategies, such as reducing fertilizer use in agriculture and improving wastewater treatment, are crucial for maintaining healthy estuarine ecosystems. For instance, the Chesapeake Bay Program has implemented various initiatives to reduce nutrient pollution, aiming to improve water quality and restore the health of the bay’s ecosystem.
How Human Activities Affect the Estuarine Food Chain
Human activities have a significant and often detrimental impact on estuarine food chains. Pollution, habitat destruction, and overfishing are major drivers of these impacts, leading to shifts in species composition, reduced biodiversity, and decreased ecosystem resilience.Pollution from various sources poses a significant threat:
- Industrial and municipal waste: Can introduce toxic chemicals, heavy metals, and organic pollutants that can accumulate in the food web, affecting organisms at all trophic levels.
- Agricultural runoff: Contributes excess nutrients, pesticides, and herbicides that can disrupt the food web as described above.
- Oil spills: Can directly kill organisms, contaminate habitats, and disrupt feeding behaviors.
- Plastic pollution: Microplastics and larger debris can be ingested by organisms, leading to physical harm and the transfer of pollutants through the food web.
Habitat destruction, including the loss of salt marshes, seagrass beds, and mangrove forests, eliminates critical habitats for many estuarine organisms. These habitats provide:
- Nursery grounds: Many fish and invertebrate species use these habitats for spawning and juvenile development.
- Shelter from predators: Provides protection from predation.
- Food sources: Support food chains, providing organic matter and detritus.
Overfishing can disrupt the balance of the food web by removing top predators or targeting key prey species. This can lead to:
- Trophic cascades: Changes in the abundance of one species can ripple through the food web, affecting other species at different trophic levels.
- Reduced biodiversity: Selective fishing can lead to a decline in the abundance of certain species, reducing the overall diversity of the ecosystem.
- Changes in size structure: Overfishing often leads to smaller average sizes of fish, affecting reproductive output and overall population health.
The combined effects of these human activities can result in significant changes in the structure and function of estuarine food webs, impacting the health and sustainability of these valuable ecosystems. For example, the decline of oyster reefs due to habitat destruction, pollution, and overfishing has had cascading effects on the Chesapeake Bay ecosystem, impacting water quality, fisheries, and overall biodiversity.
Restoration efforts, such as habitat restoration and sustainable fishing practices, are essential for mitigating these impacts and protecting estuarine ecosystems.
Estuarine Food Web Interactions: Estuarine Food Chain
Estuarine food webs are intricate networks of interacting organisms, where energy and nutrients flow through various trophic levels. Understanding these interactions is crucial for comprehending the overall health and stability of these ecosystems. These interactions involve a variety of relationships, from direct predator-prey dynamics to more complex symbiotic associations and the disruptive influence of external factors like invasive species.
Symbiotic Relationships in the Estuarine Food Web
Symbiotic relationships, encompassing mutualism, commensalism, and parasitism, are common and significantly impact estuarine food web dynamics. These interactions can influence nutrient cycling, habitat structure, and the overall biodiversity of the ecosystem.
- Mutualism: This type of symbiosis benefits both interacting species. A prime example is the relationship between certain species of mangrove trees and the various invertebrates that colonize their roots. The mangroves provide habitat and shelter, while the invertebrates, such as certain crabs and barnacles, help aerate the sediment around the roots and contribute to nutrient cycling through their waste products.
Another example involves the cleaner shrimp and larger fish. The shrimp consume parasites and dead tissue from the fish, providing a cleaning service while gaining a food source.
- Commensalism: In commensal relationships, one species benefits while the other is neither harmed nor helped. Examples include the association between certain filter-feeding organisms and the sessile invertebrates that provide them with a substrate to attach to. Filter feeders, like some species of barnacles, benefit from the availability of a stable surface without significantly affecting the host organism. Another example is the relationship between oyster reefs and various species of fish and invertebrates.
The oyster reefs provide a complex three-dimensional habitat, offering shelter and protection to the fish and invertebrates without directly benefiting or harming the oysters themselves.
- Parasitism: Parasitism involves one species (the parasite) benefiting at the expense of another (the host). Parasites in estuarine environments can range from microscopic protozoa to larger organisms like parasitic worms. For instance, the parasitic isopod
-Lironeca ovalis* infests the mouths of various fish species, feeding on their blood and tissues, weakening the host and potentially impacting its survival and reproductive success.Similarly, various trematode parasites utilize estuarine snails as intermediate hosts before infecting higher-trophic-level organisms like birds.
Effects of Invasive Species on Estuarine Food Webs
Invasive species pose a significant threat to estuarine ecosystems, often disrupting established food web dynamics through competition, predation, and habitat alteration. Their introduction can lead to substantial shifts in species composition and ecosystem function.
- Competition: Invasive species can outcompete native organisms for resources such as food and space. The European green crab (*Carcinus maenas*), for example, is a highly successful invader in many estuaries worldwide. It competes directly with native crab species and other benthic invertebrates for food and shelter, leading to declines in native populations. The rapid proliferation of invasive plants, such as
-Phragmites australis* (common reed), can outcompete native vegetation, altering habitat structure and reducing the availability of resources for native herbivores and other organisms. - Predation: Some invasive species are effective predators that can decimate native populations. The zebra mussel (*Dreissena polymorpha*), though not typically considered a direct predator, significantly alters food webs by consuming large quantities of phytoplankton, thus reducing food availability for native filter feeders and impacting higher trophic levels that rely on them. The introduction of the Asian shore crab (*Hemigrapsus sanguineus*) has been linked to declines in native invertebrate populations in some estuaries due to its aggressive predatory behavior.
- Habitat Alteration: Invasive species can physically alter habitats, impacting the organisms that depend on them. The rapid growth of
-Phragmites australis* forms dense monocultures that can displace native marsh vegetation, altering the physical structure of the habitat and reducing biodiversity. The burrowing activity of the nutria (*Myocastor coypus*), an invasive rodent, can destabilize marsh banks, leading to erosion and habitat loss, thereby affecting various organisms.
Comparison of Food Web Structures in Different Types of Estuaries
The structure of food webs varies significantly across different types of estuaries, primarily due to variations in salinity gradients, tidal influences, and substrate characteristics. These factors influence the distribution and abundance of organisms, leading to distinct food web configurations.
- Salt Wedge Estuaries: These estuaries are characterized by a strong salinity gradient, with freshwater flowing over a denser saltwater wedge. Food webs in salt wedge estuaries often have a high proportion of planktonic primary producers. The abundance of phytoplankton supports a diverse zooplankton community, which in turn supports higher trophic levels like fish and invertebrates. Examples include the Mississippi River delta and the Columbia River estuary.
- Partially Mixed Estuaries: These estuaries exhibit a more moderate salinity gradient, with some mixing between freshwater and saltwater. They often support a diverse community of both planktonic and benthic primary producers. The food webs are characterized by a mix of pelagic and benthic pathways, with detritus playing a significant role in energy flow. Examples include the Chesapeake Bay and the Delaware Bay.
- Well-Mixed Estuaries: These estuaries experience strong tidal mixing, resulting in a relatively uniform salinity throughout the water column. Benthic primary production, such as seagrass beds and macroalgae, often plays a crucial role in these food webs. Detritus derived from these primary producers fuels a complex benthic food web, supporting a diverse community of invertebrates and fish. Examples include many of the estuaries along the coast of the United Kingdom and the North Sea.
- Fjords: Fjords are characterized by deep, narrow basins with a shallow sill at the mouth, restricting water exchange. These estuaries often have low oxygen levels in the deeper waters, influencing the distribution of organisms and the structure of the food web. Detritus from the surface waters sinks to the bottom, supporting a benthic food web that is often dominated by deposit feeders and scavengers.
Methods for Studying Estuarine Food Chains
Understanding the intricate relationships within estuarine food chains requires employing a diverse array of scientific methodologies. These methods allow researchers to identify the organisms present, determine their feeding habits, and quantify the flow of energy through the ecosystem. By integrating various techniques, a comprehensive understanding of estuarine food web dynamics can be achieved, aiding in effective conservation and management strategies.
Methods for Studying Estuarine Food Webs
Researchers utilize a variety of techniques to unravel the complexities of estuarine food webs. These methods provide crucial insights into the structure and function of these dynamic ecosystems.
- Gut Content Analysis: This method involves examining the stomach contents of organisms to identify what they have recently consumed. The process typically includes:
- Collection: Specimens are collected from the field, and the digestive tracts are carefully removed.
- Dissection: The gut is opened, and the contents are examined under a microscope.
- Identification: Prey items are identified to the lowest taxonomic level possible, often using morphological characteristics like scales, spines, or skeletal structures.
- Quantification: The abundance of each prey item is estimated, either by counting the number of individuals or by estimating the volume or weight of each item.
Gut content analysis provides a direct measure of an organism’s diet at a specific point in time. However, it offers a “snapshot” of recent feeding and can be limited by the rate of digestion, which varies among species and prey types. For instance, a study of the Atlantic menhaden ( Brevoortia tyrannus) revealed their diet predominantly consisted of phytoplankton and zooplankton, showcasing their role as primary consumers in the estuarine ecosystem.
- Stable Isotope Analysis: This technique uses the ratios of stable isotopes of elements like carbon ( 13C/ 12C) and nitrogen ( 15N/ 14N) to trace the flow of energy through the food web. The process works because organisms incorporate the isotopic signature of their food into their tissues.
- Sample Collection: Tissue samples (e.g., muscle, fin clips) are collected from various organisms in the food web.
- Sample Preparation: Samples are dried, homogenized, and analyzed using an isotope ratio mass spectrometer.
- Data Analysis: The isotopic ratios are compared across different trophic levels. Organisms with higher 15N/ 14N ratios typically occupy higher trophic levels.
Stable isotope analysis provides a time-integrated view of an organism’s diet, reflecting its feeding habits over a longer period than gut content analysis. It is particularly useful for identifying the primary sources of energy supporting the food web. For example, a study in the Chesapeake Bay used stable isotope analysis to show that the dominant energy source for oysters was a mixture of phytoplankton and detritus, revealing the importance of both in supporting the oyster population.
- Fatty Acid Analysis: Fatty acids are essential components of cell membranes and play crucial roles in energy storage and signaling. Analysis of fatty acid profiles in organisms can provide insights into their dietary sources.
- Sample Collection and Preparation: Similar to stable isotope analysis, tissue samples are collected, dried, and processed.
- Extraction and Analysis: Lipids are extracted from the tissue, and the fatty acids are separated and identified using gas chromatography.
- Data Interpretation: The relative proportions of different fatty acids in an organism’s tissues are compared to those found in potential food sources.
This method is particularly useful for tracking the flow of specific dietary components, such as essential fatty acids, through the food web. For instance, analyzing fatty acid profiles can help determine the importance of specific algal species in supporting the growth and reproduction of zooplankton in an estuary.
- Molecular Techniques (DNA Barcoding and Metabarcoding): These techniques utilize DNA to identify prey items in the diet of consumers.
- Sample Collection: Fecal samples or gut contents are collected.
- DNA Extraction and Amplification: DNA is extracted from the samples, and specific gene regions (e.g., the COI gene for DNA barcoding) are amplified using PCR.
- Sequencing and Identification: The amplified DNA is sequenced, and the resulting sequences are compared to a reference database to identify the prey species.
DNA barcoding can identify prey items to the species level, even when morphological identification is difficult due to digestion. Metabarcoding, which involves sequencing all DNA present in a sample, can provide a comprehensive overview of the entire diet. For example, analyzing the feces of seabirds in an estuary using DNA barcoding can identify the specific fish species they are consuming, providing insights into their foraging behavior.
Ecological Modeling in Understanding Estuarine Food Webs, Estuarine food chain
Ecological modeling is a powerful tool for simulating and understanding the complex interactions within estuarine food webs. These models can incorporate various factors, including species interactions, environmental conditions, and nutrient dynamics, to predict the consequences of changes in the ecosystem.
- Types of Models:
- Trophic Models: These models focus on the flow of energy and nutrients through the food web, often representing different trophic levels and their interactions. Examples include Ecopath with Ecosim, which uses a mass-balance approach to estimate energy flow and simulate the effects of fishing or other disturbances.
- Individual-Based Models (IBMs): IBMs simulate the behavior and interactions of individual organisms, allowing researchers to explore how these individual-level processes influence population dynamics and food web structure. These models can incorporate complex behaviors, such as foraging strategies and predator-prey interactions.
- Dynamic Models: Dynamic models simulate the changes in population sizes and other ecosystem variables over time, often using differential equations to represent the rates of change. These models can be used to predict the effects of environmental changes or management interventions on the food web.
- Model Development: The process of building an ecological model involves several steps:
- Conceptualization: Defining the scope of the model, identifying the key components of the food web, and specifying the interactions between them.
- Data Collection: Gathering data on species abundance, diet composition, growth rates, and other relevant parameters.
- Model Parameterization: Estimating the values of the parameters in the model using data from the field or laboratory.
- Model Calibration and Validation: Adjusting the model parameters to fit the observed data and testing the model’s ability to predict real-world outcomes.
- Model Application: Using the model to simulate different scenarios, such as changes in fishing pressure or nutrient inputs, and predicting their effects on the food web.
- Model Applications: Ecological models are used to:
- Assess the Impacts of Fishing: Models can be used to evaluate the effects of different fishing strategies on target species and the broader food web, helping to inform sustainable fisheries management.
- Predict the Effects of Climate Change: Models can incorporate the effects of temperature changes, sea-level rise, and ocean acidification on estuarine ecosystems, allowing researchers to predict how these changes will impact food web structure and function.
- Evaluate the Effectiveness of Management Actions: Models can be used to assess the potential impacts of management interventions, such as habitat restoration or nutrient reduction programs, on the health and resilience of estuarine ecosystems.
Conducting a Field Study to Investigate the Diet of a Specific Estuarine Species
Conducting a field study to investigate the diet of a specific estuarine species requires careful planning, execution, and data analysis. The following steps Artikel the process:
- Species Selection and Research Question:
- Choose a target species based on its ecological importance, conservation status, or research interest.
- Formulate a clear and specific research question, such as “What is the primary diet of the species X in estuary Y?” or “How does the diet of species X vary with habitat type?”
- Study Site Selection and Sampling Design:
- Select a study site that is representative of the species’ habitat and accessible for sampling.
- Develop a sampling design that ensures sufficient sample size and statistical power. Consider factors like:
- Sampling Method: Choose an appropriate method for capturing the target species (e.g., seine nets, traps, angling).
- Sampling Frequency: Determine the frequency of sampling (e.g., daily, weekly, monthly) based on the research question and the species’ life history.
- Replicates: Include replicates to account for natural variability and increase the statistical power of the study.
- Field Data Collection:
- Capture individuals of the target species.
- Record relevant data for each individual, such as:
- Size: Measure the length and weight of the individual.
- Location: Record the location where the individual was captured.
- Date and Time: Note the date and time of capture.
- Collect samples for diet analysis:
- Gut Content Analysis: Remove the digestive tract and preserve the contents (e.g., in formalin or ethanol).
- Stable Isotope Analysis: Collect tissue samples (e.g., muscle or fin clips) and preserve them.
- Other Methods: Collect samples for fatty acid analysis or molecular techniques, as needed.
- Laboratory Analysis:
- Process the collected samples using the chosen methods:
- Gut Content Analysis: Identify and quantify prey items under a microscope.
- Stable Isotope Analysis: Analyze the isotopic ratios in the tissue samples using an isotope ratio mass spectrometer.
- Other Methods: Perform the necessary laboratory procedures for fatty acid analysis or molecular techniques.
- Process the collected samples using the chosen methods:
- Data Analysis and Interpretation:
- Analyze the data to answer the research question:
- Gut Content Analysis: Calculate the frequency of occurrence, percent composition, and index of relative importance of different prey items.
- Stable Isotope Analysis: Compare the isotopic signatures of the target species to those of potential food sources.
- Other Methods: Analyze the data from fatty acid analysis or molecular techniques.
- Interpret the results in the context of the species’ ecology and the estuarine environment.
- Assess the statistical significance of any observed patterns or trends.
- Analyze the data to answer the research question:
- Reporting and Dissemination:
- Prepare a written report or manuscript summarizing the study’s methods, results, and conclusions.
- Disseminate the findings through scientific publications, presentations, or outreach activities.
Final Conclusion
In the grand narrative of the estuarine food chain, we’ve explored a world where every link, from the sun’s embrace to the decomposers’ work, contributes to a thriving ecosystem. From the primary producers to the apex predators, each plays a crucial role in the intricate dance of life. As we conclude, let us remember the importance of protecting these invaluable ecosystems, ensuring that future generations can witness the beauty and abundance of the estuarine food chain, a testament to nature’s resilience and interconnectedness.
Let us be guardians, for the health of our estuaries reflects the health of our planet.