Food chain algae are the unsung heroes of aquatic and, to a lesser extent, terrestrial ecosystems, forming the foundational level of countless food webs. These microscopic organisms, representing diverse types of algae, are the primary producers, converting sunlight into energy through photosynthesis. Their existence fuels the survival of countless species, from tiny herbivores to apex predators, making them critical indicators of ecosystem health.
This exploration will delve into the intricacies of algae’s role, examining their photosynthetic prowess, the environmental factors governing their growth, and the intricate relationships they forge with herbivores and carnivores. We’ll analyze the impacts of nutrient availability, pollution, and climate change on algal populations, along with human interactions, including algae’s potential as a biofuel source and its use in food and other products.
Visual aids, such as illustrations and diagrams, will further illuminate the complex dynamics of algal-based food chains.
Introduction to Food Chain Algae
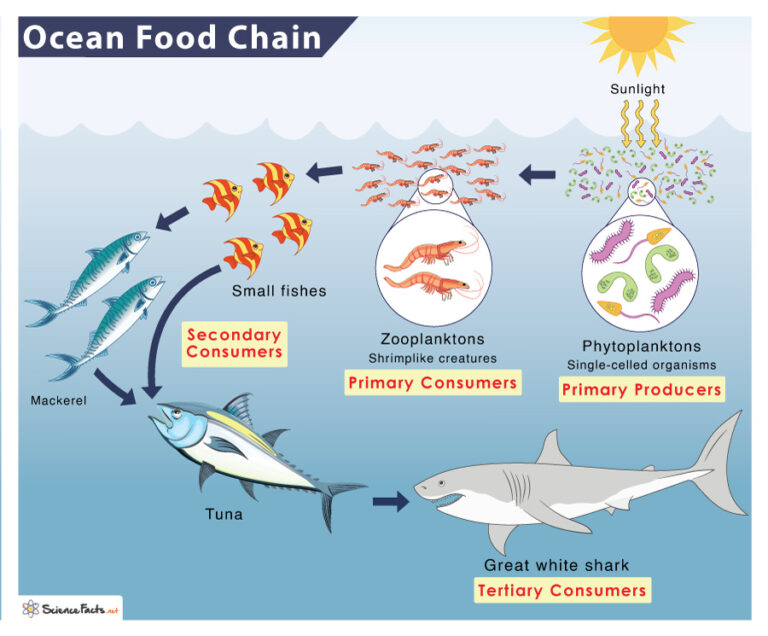
Algae are fundamental organisms that underpin the structure and function of countless ecosystems. Their significance stems from their role as primary producers, converting inorganic substances into organic matter through photosynthesis. This process fuels the entire food web, making algae crucial for the survival of diverse species, from microscopic zooplankton to large marine mammals. Understanding algae is key to comprehending the health and dynamics of our planet’s aquatic and terrestrial environments.Algae represent a diverse group of photosynthetic organisms, ranging from single-celled microscopic forms to large, multicellular seaweeds.
They are found in almost every environment on Earth, from oceans and freshwater lakes to damp soil and even snow. Their diversity extends beyond size and form, encompassing a wide array of pigments and metabolic strategies.
The Fundamental Role of Algae in Aquatic and Terrestrial Food Webs
Algae are the foundation of most aquatic food webs, serving as the primary producers that convert sunlight into energy. This energy, stored in the form of sugars, fuels the growth and reproduction of algae, and is then transferred to other organisms through consumption. This process is essential for maintaining the balance of ecosystems and supporting biodiversity. In terrestrial environments, while not as dominant as in aquatic systems, algae still contribute significantly, particularly in moist habitats and as a component of lichens.
- Aquatic Food Webs: In oceans, lakes, and rivers, phytoplankton (microscopic algae) form the base of the food web. These organisms are consumed by zooplankton, which in turn are eaten by small fish, and so on up the food chain. For example, in the North Atlantic, diatoms, a type of phytoplankton, are the primary food source for copepods, small crustaceans that are then consumed by fish like herring, which are preyed upon by larger predators such as seals and whales.
The health of these entire food webs depends on the productivity of the algae.
- Terrestrial Food Webs: While less prevalent, algae play a role in terrestrial ecosystems. They contribute to the formation of soil crusts in arid environments and are a key component of lichens, symbiotic organisms composed of algae and fungi. These lichens provide food and shelter for various invertebrates and contribute to soil formation. Furthermore, algae can colonize damp surfaces, providing a food source for small herbivores and detritivores.
- Energy Transfer and Biomass Production: Algae efficiently convert sunlight into biomass through photosynthesis. This biomass is then consumed and transferred up the food chain, supporting the growth and survival of other organisms. The amount of energy that algae can fix determines the carrying capacity of the ecosystem.
Overview of Different Types of Algae
The classification of algae is complex, reflecting their evolutionary diversity. They are grouped based on their cellular structure, pigments, and other characteristics. Understanding these different types is crucial for appreciating the variety of ecological roles they play.
- Green Algae (Chlorophyta): These algae are characterized by the presence of chlorophyll a and b, giving them a green color. They are found in freshwater, marine, and terrestrial environments. Examples include Chlamydomonas (a single-celled alga) and Ulva (sea lettuce).
- Brown Algae (Phaeophyceae): Brown algae, including kelp and other seaweeds, are primarily found in marine environments. They contain chlorophyll a, chlorophyll c, and fucoxanthin, which gives them their characteristic brown color. Kelp forests are important habitats for many marine species.
- Red Algae (Rhodophyta): Red algae are mostly marine and contain chlorophyll a, phycoerythrin, and phycocyanin, giving them a red color. They are often found in deeper waters where they can absorb the blue-green light that penetrates the ocean.
- Diatoms (Bacillariophyceae): Diatoms are single-celled algae with intricate silica cell walls. They are found in both marine and freshwater environments and are a major component of phytoplankton.
- Dinoflagellates (Dinophyceae): These single-celled algae are characterized by two flagella and are often bioluminescent. Some species can cause harmful algal blooms, also known as red tides.
Significance of Algae as Primary Producers
Algae are the foundation of aquatic ecosystems and are vital to the global carbon cycle. Their photosynthetic activity supports almost all life in these environments.
- Photosynthesis and Oxygen Production: Algae, through photosynthesis, convert carbon dioxide and water into organic compounds, using sunlight as an energy source. A significant portion of the oxygen in Earth’s atmosphere is produced by algae, particularly phytoplankton in the oceans.
6CO₂ + 6H₂O + Light Energy → C₆H₁₂O₆ + 6O₂
This equation illustrates the fundamental process of photosynthesis, showing the conversion of carbon dioxide and water into glucose (sugar) and oxygen.
- Carbon Sequestration: Algae absorb atmospheric carbon dioxide during photosynthesis, helping to regulate the Earth’s climate. When algae die, some of the carbon they have fixed can be stored in sediments, effectively removing it from the atmosphere for extended periods. This process is crucial for mitigating climate change.
- Support for Aquatic Food Webs: As primary producers, algae provide the energy and nutrients that sustain all other organisms in aquatic food webs. Their abundance and productivity directly influence the health and diversity of these ecosystems.
- Biomass Production and Ecosystem Services: Algae produce a substantial amount of biomass, forming the base of the food web. They also provide other ecosystem services, such as habitat for various organisms and the cycling of nutrients. For instance, kelp forests provide shelter and food for numerous marine species, contributing to the overall health and resilience of coastal ecosystems.
Algae as Primary Producers
Algae form the foundation of many aquatic food webs, acting as the primary producers that convert light energy into chemical energy. Their photosynthetic activity fuels ecosystems, supporting a diverse range of organisms. Understanding their role is crucial for comprehending the intricate dynamics of these environments.
For descriptions on additional topics like low sodium fast food pdf, please visit the available low sodium fast food pdf.
Photosynthesis in Algae
Photosynthesis is the fundamental process by which algae convert light energy into chemical energy in the form of glucose, their primary food source. This process is essential for life on Earth, as it provides the oxygen we breathe and forms the base of most food chains.The process can be summarized as follows:
CO2 + H 2O + Light Energy → C 6H 12O 6 + O 2
Algae possess various photosynthetic pigments, including chlorophylls and accessory pigments like carotenoids and phycobilins, that capture light energy at different wavelengths. These pigments enable algae to thrive in diverse light environments, from the surface of water bodies to deeper depths where light penetration is limited. The efficiency of photosynthesis varies depending on the algal species and environmental conditions. For example, the specific type of chlorophyll present (e.g., chlorophyll a, b, c, or d) and the presence of accessory pigments will influence the light wavelengths absorbed and thus, the photosynthetic rate.
This is a crucial aspect for understanding the varying ecological niches algae occupy.
Environmental Factors Affecting Algal Growth and Productivity
Algal growth and productivity are influenced by a complex interplay of environmental factors. These factors can be broadly categorized into light availability, nutrient availability, temperature, salinity, and water movement. Understanding these factors is critical for predicting algal blooms, managing aquatic ecosystems, and even cultivating algae for various applications.Here’s a look at how these factors affect algae:
- Light Availability: Light is essential for photosynthesis. The intensity and quality of light influence the rate of photosynthesis. Algae near the surface receive more light, but excessive light can also inhibit photosynthesis due to photoinhibition. The depth to which light penetrates water is also critical, influencing the vertical distribution of algal species.
- Nutrient Availability: Nutrients like nitrogen (N), phosphorus (P), and silicon (Si) are essential for algal growth. Nutrient availability often limits algal growth, especially in freshwater and marine environments. Eutrophication, the excessive enrichment of water bodies with nutrients, can lead to algal blooms, which can deplete oxygen and harm aquatic life.
- Temperature: Temperature affects the rate of biochemical reactions involved in photosynthesis and other metabolic processes. Different algal species have different optimal temperature ranges. Generally, warmer temperatures accelerate growth up to a certain point, after which excessive heat can be detrimental.
- Salinity: Salinity, the salt content of water, affects the osmotic balance of algal cells. Different algal species have varying tolerances to salinity. Freshwater algae are adapted to low salinity, while marine algae are adapted to high salinity. Changes in salinity can stress algae and affect their growth.
- Water Movement: Water movement influences nutrient availability, gas exchange, and light exposure. Water currents can bring nutrients to algae and remove waste products. Mixing of water layers can also expose algae to light at different depths. Excessive water turbulence can damage fragile algal cells.
Photosynthetic Efficiency of Different Algal Species
The photosynthetic efficiency of algae varies significantly among different species due to differences in pigment composition, cellular structure, and environmental adaptations. Comparing the photosynthetic efficiency of various algal species provides insights into their ecological niches and potential applications.Here’s a table summarizing the photosynthetic efficiency of selected algal species:
Algal Species | Photosynthetic Pigments | Optimal Light Intensity (µmol photons/m²/s) | Maximum Photosynthetic Rate (mg O2/mg Chl a/hr) |
---|---|---|---|
Chlorella vulgaris (Green Algae) | Chlorophyll a, Chlorophyll b, Carotenoids | 100-200 | 40-60 |
Spirulina platensis (Blue-Green Algae/Cyanobacteria) | Chlorophyll a, Phycocyanin, Carotenoids | 50-150 | 25-40 |
Dunaliella salina (Green Algae) | Chlorophyll a, Beta-carotene, Carotenoids | 200-400 | 30-50 |
Phaeodactylum tricornutum (Diatom) | Chlorophyll a, Chlorophyll c, Fucoxanthin | 150-300 | 35-55 |
This table illustrates how different algal species have adapted to varying light intensities and exhibit different photosynthetic rates.
- Chlorella vulgaris*, for instance, demonstrates a relatively high photosynthetic rate under moderate light conditions, making it a popular choice for biofuel production.
- Spirulina platensis*, adapted to high-salinity environments, exhibits lower photosynthetic rates but thrives in challenging conditions.
- Dunaliella salina* is highly efficient in producing beta-carotene, which protects it from intense light.
- Phaeodactylum tricornutum*, a diatom, has a photosynthetic rate comparable to green algae but utilizes different accessory pigments. These differences highlight the diversity of algal adaptations and their ecological significance.
Algae and Herbivores
The intricate dance between algae and herbivores is a fundamental aspect of aquatic and even some terrestrial ecosystems. Herbivores, the primary consumers, rely on algae as their main food source, shaping algal populations and influencing the overall health and structure of these environments. Understanding this relationship is crucial for comprehending the dynamics of food webs and the impact of environmental changes.
Herbivores that Consume Algae
A diverse range of organisms, spanning various taxonomic groups, have evolved to feed on algae. These herbivores play a significant role in regulating algal growth and biomass.
- Zooplankton: Microscopic animals, such as copepods and cladocerans (e.g., Daphnia), are common grazers in freshwater and marine environments. They consume phytoplankton, including various types of algae.
- Invertebrates: Many invertebrate species, including snails (gastropods), sea urchins (echinoderms), and various insect larvae, are specialized algal grazers. Sea urchins, for example, are key herbivores in kelp forests, where they can significantly impact the abundance of kelp.
- Fish: Several fish species, such as parrotfish and surgeonfish in coral reefs, and some freshwater fish like certain types of carp, are adapted to consume algae. Their grazing activities can influence the distribution and composition of algal communities.
- Marine Mammals: The West Indian manatee ( Trichechus manatus) is a large herbivorous marine mammal that primarily feeds on aquatic plants, including algae, in shallow coastal waters.
Adaptations of Herbivores to Consume Algae
Herbivores have developed a variety of adaptations to efficiently harvest and digest algae. These adaptations reflect the diverse forms and characteristics of algae, as well as the environments in which herbivores live.
- Specialized Mouthparts: Many herbivores possess specialized mouthparts designed for scraping, rasping, or filtering algae. For instance, snails have a radula, a tongue-like structure with tiny teeth, used to scrape algae from surfaces. Parrotfish have beak-like mouths and strong teeth to scrape algae from coral.
- Digestive Systems: The digestive systems of herbivores are often adapted to break down the complex cell walls of algae and extract nutrients efficiently. Some herbivores have specialized enzymes or symbiotic microorganisms in their guts to aid in digestion. For example, some fish species have long, coiled intestines to increase the surface area for nutrient absorption.
- Filter Feeding Mechanisms: Some herbivores, such as certain zooplankton, are filter feeders. They use specialized appendages to filter algae from the water column. Copepods, for example, have setae (bristle-like structures) on their feeding appendages that trap algal cells.
- Behavioral Adaptations: Herbivores also exhibit behavioral adaptations to optimize their foraging efficiency. They may actively search for algae, select specific algal types, or graze in coordinated groups. Sea urchins, for instance, often move in groups to maximize their grazing impact on kelp forests.
Impacts of Herbivore Grazing on Algal Populations
Herbivore grazing significantly influences algal populations, affecting their abundance, distribution, and community structure. The intensity of grazing pressure can vary depending on the herbivore species, their density, and environmental conditions.
- Population Control: Herbivores can effectively control algal populations, preventing excessive algal blooms. In environments with high herbivore densities, algae may be kept at relatively low levels.
- Community Structure: Grazing can alter the composition of algal communities. Herbivores may preferentially consume certain algal species, leading to shifts in the relative abundance of different algae. This can influence the overall diversity and stability of the algal community.
- Nutrient Cycling: Herbivores play a role in nutrient cycling by consuming algae and releasing nutrients back into the environment through excretion. This can influence the availability of nutrients for algal growth.
- Habitat Modification: In some ecosystems, herbivore grazing can modify the physical structure of the environment. For example, in kelp forests, intense grazing by sea urchins can lead to the overgrazing of kelp, creating “urchin barrens” where the habitat is drastically altered.
- Trophic Cascades: Herbivore grazing can trigger trophic cascades, where the effects of grazing ripple through the food web. For example, the removal of herbivores can lead to increased algal growth, which can, in turn, affect other organisms in the ecosystem.
Algae and Carnivores
Carnivores, the meat-eaters of the aquatic world, play a crucial role in algal-based food chains. They occupy the higher trophic levels, obtaining energy indirectly from algae through a series of predator-prey interactions. Their presence significantly influences the structure and dynamics of these ecosystems, maintaining balance and preventing algal blooms from spiraling out of control.
Energy Transfer from Algae to Carnivores
The transfer of energy from algae to carnivores is a multi-step process, intricately woven through the food web. Algae, as primary producers, capture solar energy through photosynthesis, converting it into chemical energy stored in organic compounds. This energy is then passed on as herbivores consume algae, and subsequently, carnivores consume herbivores. The carnivores, in turn, obtain their energy by consuming other animals, ultimately tracing back to the initial energy captured by the algae.
This process follows the fundamental principle of energy flow in ecosystems, where energy decreases with each successive trophic level due to metabolic processes and the inefficiency of energy transfer.
Carnivores’ Role in Regulating Algal Populations
Carnivores exert a significant top-down control on algal populations, influencing their abundance and distribution. By preying on herbivores, carnivores limit the grazing pressure on algae, preventing unchecked algal growth. This indirect control can lead to a more stable and diverse ecosystem. For example, consider a scenario where a lake experiences a surge in algal growth, potentially leading to an algal bloom.
If the lake ecosystem has a healthy population of carnivorous fish that prey on herbivorous zooplankton (which feed on algae), the carnivores can reduce the zooplankton population. This, in turn, decreases the grazing pressure on the algae, helping to control the bloom.
Trophic Levels in an Algal-Based Food Chain
The following bulleted list Artikels the various trophic levels typically involved in an algal-based food chain, illustrating the flow of energy and the relationships between organisms:
- Primary Producers: Algae (e.g., diatoms, green algae, cyanobacteria) – These organisms use sunlight to produce their own food through photosynthesis, forming the base of the food chain. They are the foundation of the ecosystem, converting inorganic substances into organic matter.
- Primary Consumers (Herbivores): Herbivores, like zooplankton (e.g., copepods, water fleas), aquatic insect larvae, and some small fish, directly consume algae. They obtain energy by grazing on the primary producers.
- Secondary Consumers (Carnivores): These organisms, like small fish, some crustaceans, and aquatic insects, consume the primary consumers (herbivores). They are the first level of carnivores in the food chain.
- Tertiary Consumers (Apex Predators): Apex predators, such as larger fish, birds, and mammals, occupy the highest trophic level in the chain. They feed on secondary consumers and sometimes other carnivores, playing a crucial role in regulating the entire ecosystem’s structure.
- Decomposers: Decomposers, such as bacteria and fungi, break down dead organic matter from all trophic levels, returning essential nutrients to the ecosystem. They play a vital role in nutrient cycling, making these nutrients available for the primary producers, algae, to use again.
Factors Affecting Algae Populations: Food Chain Algae
Algae populations, crucial components of aquatic ecosystems, are highly sensitive to various environmental factors. Understanding these factors is critical for predicting algal bloom occurrences, assessing water quality, and managing the health of aquatic environments. This section will explore the significant influences on algae populations, including nutrient availability, pollution, and climate change.
Impact of Nutrient Availability on Algal Blooms
Nutrient availability is a primary driver of algal population dynamics. Excess nutrients, particularly nitrogen and phosphorus, can lead to explosive algal growth, known as algal blooms.The availability of nutrients directly impacts algal growth rates.
Algae, like plants, require nutrients for photosynthesis and cellular processes.
When nutrients are abundant, algae can rapidly multiply, leading to increased biomass and the potential for blooms.
- Eutrophication: This process, characterized by excessive nutrient enrichment, is a major cause of algal blooms. Sources of these nutrients include agricultural runoff (fertilizers), sewage discharge, and industrial waste. Eutrophication can transform clear waters into murky, algae-dominated systems.
- Nitrogen and Phosphorus: These are often the limiting nutrients in aquatic ecosystems. The availability of nitrogen and phosphorus dictates the rate of algal growth. For instance, in freshwater lakes, phosphorus is often the limiting nutrient, while in marine environments, nitrogen may be the primary limiting factor. When these nutrients become overly abundant, they trigger bloom events.
- Bloom Characteristics: Algal blooms can manifest in various ways, from visible surface scums to changes in water color. Some blooms are harmless, while others produce toxins (harmful algal blooms or HABs) that can be dangerous to humans and wildlife. HABs can lead to fish kills, shellfish poisoning, and other ecological and health problems.
- Examples: The Great Lakes region of North America has experienced frequent algal blooms due to agricultural runoff and other sources of nutrient pollution. Similarly, the Gulf of Mexico experiences a “dead zone” each year, caused by excessive nutrient input from the Mississippi River, fueling algal blooms that deplete oxygen levels in the water.
Effects of Pollution on Algal Growth and Diversity
Pollution significantly affects algae, impacting both their growth rates and the diversity of algal communities. Various pollutants can disrupt algal physiology and alter the structure and function of aquatic ecosystems.Different types of pollutants exert different effects on algae. Some pollutants can directly inhibit growth, while others can alter the competitive balance among different algal species.
- Toxic Substances: Heavy metals (e.g., mercury, lead), pesticides, and herbicides can be toxic to algae, inhibiting their growth or causing cell damage. These pollutants can bioaccumulate in algae and move up the food chain, affecting other organisms.
- Organic Pollutants: Sewage and industrial waste can introduce organic matter into aquatic systems. While some organic matter can serve as a nutrient source, excessive amounts can lead to oxygen depletion as bacteria decompose the organic material, harming algae and other aquatic life.
- Oil Spills: Oil spills can coat algal cells, interfering with their ability to photosynthesize and absorb nutrients. Oil can also release toxic compounds that harm algae.
- Impact on Diversity: Pollution can reduce algal diversity. Sensitive species may be eliminated, while more tolerant species may thrive, leading to a shift in the composition of algal communities. This can have cascading effects on the entire ecosystem, affecting the organisms that rely on algae as a food source.
- Example: The Deepwater Horizon oil spill in the Gulf of Mexico had significant impacts on phytoplankton communities, with some species experiencing mortality and changes in community composition.
Comparison of Climate Change Effects on Different Types of Algae
Climate change is altering aquatic environments globally, affecting algae populations in various ways. The impacts of climate change vary depending on the type of algae, the geographic location, and the specific environmental changes.Different algal groups exhibit varying sensitivities to climate change-related factors. These factors include changes in temperature, ocean acidification, and altered precipitation patterns.
- Diatoms: These are single-celled algae with silica cell walls. They are important primary producers in many aquatic ecosystems.
- Temperature: Diatoms are sensitive to temperature changes, with some species thriving in colder waters and others in warmer waters. Rising water temperatures can alter the species composition of diatom communities, potentially favoring less productive species.
- Ocean Acidification: Ocean acidification, caused by increased CO2 absorption, can affect the formation of diatom cell walls, potentially reducing their growth rates.
- Dinoflagellates: These are single-celled algae, some of which are responsible for harmful algal blooms.
- Temperature: Dinoflagellates often thrive in warmer waters. Climate change is predicted to increase the frequency and intensity of dinoflagellate blooms, potentially leading to more frequent HAB events.
- Nutrient Availability: Changes in precipitation patterns and runoff can alter nutrient inputs, affecting the growth of dinoflagellates.
- Cyanobacteria (Blue-green algae): These are prokaryotic algae that can form dense blooms in freshwater and marine environments.
- Temperature: Cyanobacteria often thrive in warm, nutrient-rich waters. Rising temperatures and increased nutrient runoff are predicted to favor cyanobacterial blooms.
- Light: Cyanobacteria can tolerate low light conditions, allowing them to dominate in turbid waters.
- Seaweeds (Macroalgae): These are multicellular algae found in coastal environments.
- Temperature: Seaweeds are sensitive to temperature changes, with some species experiencing heat stress and bleaching events. Changes in temperature can also alter the distribution of seaweed species.
- Ocean Acidification: Ocean acidification can affect the calcification of some seaweed species, impacting their growth and survival.
- Overall Effects: The combined effects of climate change on algae include altered species distributions, changes in bloom frequency and intensity, and potential impacts on ecosystem productivity and food web structure.
- Example: In the Baltic Sea, warming water temperatures and increased nutrient loads have contributed to the expansion of cyanobacterial blooms, leading to oxygen depletion and other ecological problems. In coastal regions worldwide, rising sea temperatures are contributing to the decline of kelp forests, a crucial habitat for many marine species.
Types of Algae in Food Chains
Algae, the often-unseen foundation of many aquatic and even some terrestrial ecosystems, exhibit a remarkable diversity. Their presence, and the specific types that dominate, significantly shape the structure and function of food chains. Understanding the different algal groups and their ecological roles is crucial to comprehending how energy flows through these systems and how they respond to environmental changes.
Major Groups of Algae
The algal world is broadly categorized into several major groups, each with distinct characteristics and roles in food chains. These groups are differentiated by their pigments, cell wall composition, and overall structure. They each occupy different niches, contributing to the overall productivity and biodiversity of their respective ecosystems.
- Diatoms (Bacillariophyceae): Diatoms are single-celled algae characterized by their intricate silica (glass) cell walls, known as frustules. They are found in both freshwater and marine environments, and their frustules come in an astonishing array of shapes and patterns, making them a beautiful subject for microscopic study. Diatoms are a major component of phytoplankton, serving as a primary food source for many aquatic organisms.
Their abundance is often linked to nutrient availability, particularly silicon, which is essential for frustule formation.
- Green Algae (Chlorophyta): Green algae encompass a vast and diverse group, ranging from single-celled organisms to multicellular forms like sea lettuce. They are characterized by the presence of chlorophyll a and b, the same photosynthetic pigments found in land plants, giving them their characteristic green color. Green algae are found in a wide range of habitats, from freshwater lakes and rivers to the oceans and even on land.
They play a significant role as primary producers in many aquatic ecosystems, forming the base of the food chain for many herbivores.
- Dinoflagellates (Dinophyceae): Dinoflagellates are primarily single-celled organisms, often with two flagella used for movement. They are found in both marine and freshwater environments. Some dinoflagellates are photosynthetic, while others are heterotrophic, feeding on other organisms. Certain species produce toxins that can cause harmful algal blooms (HABs), also known as “red tides,” which can have devastating effects on marine life and human health.
- Brown Algae (Phaeophyceae): Brown algae are almost exclusively marine and include the large kelp forests. They are characterized by the presence of chlorophyll a and c, along with the pigment fucoxanthin, which gives them their brown color. Kelp forests provide habitat and food for a diverse array of marine organisms, playing a crucial role in coastal ecosystems.
- Red Algae (Rhodophyta): Red algae are primarily marine, though some species are found in freshwater habitats. They are characterized by the presence of chlorophyll a and phycobilins, pigments that give them their red or reddish-brown color. Red algae can thrive in deeper waters due to their ability to absorb blue light, which penetrates deeper than other wavelengths. They contribute to the primary productivity of coral reefs and other marine ecosystems.
Ecological Niches of Algal Species
The ecological niche of an algal species is defined by its role in the food chain and its interactions with the environment. This includes its habitat, its food source (if heterotrophic), its predators, and the environmental conditions it requires to thrive. Different algal species have evolved to occupy specific niches, minimizing competition and maximizing resource utilization.
- Diatoms: Diatoms typically thrive in nutrient-rich waters with ample sunlight. They are often the dominant phytoplankton species in spring blooms, exploiting the burst of nutrients released after winter. They are grazed upon by a wide range of zooplankton, such as copepods, and serve as a crucial link between primary production and higher trophic levels.
- Green Algae: Green algae occupy diverse niches. Some, like
-Chlamydomonas*, are common in freshwater ponds and lakes. Others, like
-Ulva* (sea lettuce), are found in intertidal zones, where they can tolerate fluctuating salinity and exposure to air. Their versatility allows them to colonize a wide range of habitats, supporting various herbivores. - Dinoflagellates: Dinoflagellates have diverse niches. Some are photosynthetic and thrive in the open ocean, while others are heterotrophic and feed on other organisms. Their ability to form cysts allows them to survive unfavorable conditions, such as nutrient depletion or extreme temperatures. The toxic species are particularly important, as they can impact entire food webs.
- Brown Algae: Brown algae, especially kelp, form extensive underwater forests, providing shelter and food for a vast array of marine organisms. They are adapted to cold, nutrient-rich waters and require a solid substrate for attachment. Kelp forests support complex food webs, including sea urchins, fish, and marine mammals.
- Red Algae: Red algae occupy various niches, including the deep-water habitats, as they can absorb blue light, allowing them to photosynthesize. They can also be found in intertidal zones and coral reefs. Their role in the food chain varies depending on the species; some are grazed upon directly, while others contribute to the structure of the reef or provide habitat.
Characteristics and Habitats of Different Algae Types
This table summarizes the key characteristics and habitats of the major algal groups discussed:
Algae Group | Key Characteristics | Dominant Pigments | Typical Habitats |
---|---|---|---|
Diatoms | Single-celled, silica frustules, diverse shapes | Chlorophyll a, c, and carotenoids | Marine and freshwater, nutrient-rich waters |
Green Algae | Single-celled to multicellular, diverse forms | Chlorophyll a and b | Freshwater, marine, terrestrial |
Dinoflagellates | Single-celled, often with two flagella | Chlorophyll a and c, and other pigments | Marine and freshwater |
Brown Algae | Multicellular, includes kelp, large size | Chlorophyll a and c, fucoxanthin | Marine, cold, nutrient-rich waters |
Red Algae | Mostly multicellular, various forms | Chlorophyll a, phycobilins | Marine, can thrive in deep waters |
Algae and Ecosystem Health
Algae, the often-overlooked foundation of aquatic ecosystems, serve as a critical indicator of overall environmental health. Their presence, abundance, and diversity reflect the balance and stability of the water body they inhabit. Monitoring algal populations provides valuable insights into the impacts of pollution, climate change, and other stressors on aquatic ecosystems.
Algae as Indicators of Ecosystem Health
The health of an ecosystem is intrinsically linked to the condition of its algal populations. Changes in algal community structure, such as shifts in species composition or an increase in the dominance of certain types, can signal underlying environmental problems. For instance, the presence of specific algal species can indicate the presence of pollutants like excess nutrients from agricultural runoff or sewage discharge.
A diverse and balanced algal community typically suggests a healthy ecosystem, while a community dominated by a few tolerant species might indicate degradation.
Effects of Algal Blooms on Water Quality and Aquatic Life
Algal blooms, characterized by a rapid increase in algal populations, can have significant detrimental effects on water quality and aquatic life. These blooms often result from an overabundance of nutrients, a phenomenon known as eutrophication.The consequences of algal blooms include:
- Reduced Water Clarity: Dense algal blooms cloud the water, reducing sunlight penetration. This can inhibit the growth of submerged aquatic vegetation, which provides habitat and food for other organisms.
- Oxygen Depletion: As the algae die and decompose, bacteria consume large amounts of oxygen, leading to hypoxia (low oxygen) or anoxia (no oxygen) in the water. This can suffocate fish and other aquatic animals.
- Toxin Production: Some algae, such as cyanobacteria (blue-green algae), produce toxins that can be harmful or even fatal to humans and animals. These toxins can contaminate drinking water sources and make recreational activities unsafe.
- Changes in pH: During bloom, algae can dramatically alter the pH of the water, making it too acidic or alkaline for many aquatic organisms to survive.
These impacts can cascade through the food web, affecting the entire ecosystem. For example, a fish kill caused by oxygen depletion can reduce the food supply for larger predators, leading to a decline in their populations. In extreme cases, harmful algal blooms can cause significant economic losses due to impacts on fisheries, tourism, and water treatment costs. A real-life example of this is the recurrent harmful algal blooms in Lake Erie, which have led to significant fish kills and impacted the recreational and commercial fishing industries.
Methods Used to Monitor Algal Populations
Monitoring algal populations is crucial for assessing ecosystem health and managing the impacts of algal blooms. Various methods are employed to collect data on algal abundance, species composition, and toxin production.
Here are some common methods used to monitor algal populations:
- Water Sampling: Collecting water samples at regular intervals from various locations and depths.
- Microscopic Analysis: Identifying and counting algal cells under a microscope to determine species composition and abundance.
- Pigment Analysis: Measuring the concentrations of photosynthetic pigments, such as chlorophyll a, to estimate algal biomass.
- Toxin Testing: Analyzing water samples for the presence of algal toxins using various analytical techniques, such as ELISA (enzyme-linked immunosorbent assay) or liquid chromatography-mass spectrometry (LC-MS).
- Remote Sensing: Utilizing satellite imagery and aerial photography to monitor large-scale algal blooms and track their movement. This method can measure chlorophyll concentrations across large areas.
Human Interactions with Algae
Humans have a multifaceted relationship with algae, ranging from utilizing it as a food source to grappling with the challenges posed by algal blooms. Understanding these interactions is crucial for both harnessing algae’s benefits and mitigating its negative impacts. The following sections will delve into these various aspects of human interaction with these fascinating aquatic organisms.
Algae Utilization for Food and Other Products
Algae offers a wide range of applications, extending beyond food to encompass various industrial and commercial uses. It’s a versatile resource that is increasingly recognized for its potential.
- Food Source: Certain algae species, such as spirulina and nori (used to wrap sushi), are directly consumed by humans. These algae are rich in proteins, vitamins, and minerals, making them a valuable dietary component, particularly in regions where other food sources are limited. Spirulina, for example, has a high protein content, estimated to be around 60-70% by dry weight, and is a complete protein, containing all essential amino acids.
- Animal Feed: Algae is used as a feed supplement for livestock and aquaculture. It can enhance the nutritional value of animal feed and improve animal health. For example, supplementing the diet of farmed fish with algae can increase their omega-3 fatty acid content, making them healthier for human consumption.
- Biofuel Production: Algae can be cultivated to produce biofuels, such as biodiesel and biogas. Algae’s rapid growth rate and high oil content make it a promising alternative to fossil fuels. The production of algal biofuels has the potential to reduce greenhouse gas emissions and dependence on finite resources. Research has shown that some algae species can produce significantly more oil per acre than traditional biofuel crops like soybeans or corn.
- Pharmaceuticals and Nutraceuticals: Algae is a source of various bioactive compounds used in pharmaceuticals and nutraceuticals. For instance, certain algae species produce compounds with antioxidant, anti-inflammatory, and anti-cancer properties. Astaxanthin, a carotenoid found in certain algae, is a powerful antioxidant used in supplements and cosmetics.
- Cosmetics: Algae extracts are used in cosmetic products for their moisturizing, anti-aging, and antioxidant properties. They can also act as emulsifiers and stabilizers in cosmetic formulations. The use of algae in cosmetics is growing due to its natural origin and beneficial effects on the skin.
- Fertilizers: Algae can be used as a natural fertilizer, improving soil fertility and promoting plant growth. Algal fertilizers are rich in nutrients and can enhance the water retention capacity of the soil. This application promotes sustainable agriculture by reducing the reliance on synthetic fertilizers.
Benefits and Drawbacks of Algal Farming
Algal farming, or aquaculture, offers several advantages but also presents certain challenges. A careful consideration of these aspects is crucial for sustainable and effective algae cultivation.
- Benefits:
- High Productivity: Algae can grow rapidly, producing a high biomass yield per unit area compared to traditional crops.
- Reduced Land Use: Algal farming can be conducted in various environments, including open ponds, closed photobioreactors, and even wastewater, reducing the need for arable land.
- Carbon Sequestration: Algae absorbs carbon dioxide during photosynthesis, potentially mitigating greenhouse gas emissions.
- Wastewater Treatment: Algae can remove nutrients from wastewater, such as nitrogen and phosphorus, thereby helping to purify water.
- Versatile Applications: Algae can be used for food, feed, biofuels, pharmaceuticals, and other products, offering diverse economic opportunities.
- Drawbacks:
- High Costs: The initial investment and operational costs of algal farming, particularly for closed systems, can be significant.
- Contamination Risks: Algal cultures can be susceptible to contamination by other algae species, bacteria, or viruses.
- Energy Requirements: Algal farming, especially in closed systems, can require significant energy for lighting, mixing, and temperature control.
- Water Usage: While algae can grow in wastewater, large-scale cultivation still requires substantial water resources.
- Regulation and Environmental Concerns: The environmental impact of large-scale algal farming, including the potential for nutrient runoff and ecosystem disruption, needs careful monitoring and regulation.
Methods to Mitigate the Negative Impacts of Algal Blooms on Human Activities
Algal blooms, also known as harmful algal blooms (HABs), can have significant negative impacts on human activities, including drinking water supplies, fisheries, and recreational activities. Various methods are employed to minimize these effects.
- Early Warning Systems: Monitoring programs that detect and predict algal blooms using satellite imagery, water sampling, and mathematical models can provide early warnings. These systems allow for timely intervention to protect water resources and human health.
- Nutrient Reduction: Reducing nutrient runoff from agricultural and urban sources is a key strategy. This can involve implementing best management practices, such as using fertilizers more efficiently, reducing soil erosion, and improving wastewater treatment.
- Physical Removal: Physical methods, such as skimming or filtration, can be used to remove algal blooms from water bodies. These methods are most effective for localized blooms.
- Chemical Treatments: Certain chemicals, such as algaecides, can be used to control algal blooms. However, the use of chemicals can have environmental impacts and requires careful consideration of potential side effects.
- Biological Control: Introducing organisms that prey on algae, such as certain zooplankton or viruses, can help to control algal blooms. This approach is still under development and requires careful evaluation to avoid unintended consequences.
- Water Treatment: Advanced water treatment processes, such as activated carbon filtration and ozonation, can be used to remove toxins produced by harmful algae from drinking water supplies.
- Public Education and Awareness: Educating the public about the risks associated with algal blooms and promoting responsible behaviors, such as avoiding contact with affected water, can help to protect human health.
Algae and Biofuel Production
Algae, the often-overlooked primary producers of aquatic ecosystems, are increasingly recognized for their potential beyond their ecological roles. One of the most promising applications of algae lies in biofuel production, offering a sustainable alternative to fossil fuels. This discussion will delve into the capabilities of algae in this field, exploring both its advantages and disadvantages and providing a step-by-step guide to the conversion process.
Potential of Algae as a Biofuel Source
Algae present a compelling option for biofuel production due to several advantageous characteristics. Unlike terrestrial crops, algae can grow in diverse environments, including non-arable land and saltwater, thereby minimizing competition with food production. Furthermore, certain algal species exhibit rapid growth rates, allowing for higher biomass yields per unit area compared to traditional biofuel feedstocks like corn or soybeans. This efficient growth, coupled with the potential to cultivate algae in closed systems, reduces the environmental footprint associated with land use, fertilizer application, and water consumption.
The high lipid content of many algal species, which can be converted into biodiesel or used to produce other biofuels, further enhances their appeal.
Advantages and Disadvantages of Using Algae for Biofuel Production, Food chain algae
The utilization of algae for biofuel production presents a complex set of advantages and disadvantages that must be carefully considered.
- Advantages:
- High Biomass Yields: Algae can produce significantly more biomass per acre than terrestrial crops, increasing biofuel production efficiency.
- Non-Arable Land Suitability: Algae can be cultivated on non-arable land and in saltwater, avoiding competition with food crops.
- Reduced Environmental Impact: Algae cultivation can minimize the need for fertilizers and pesticides, and some species can even absorb carbon dioxide, contributing to carbon sequestration.
- Versatile Biofuel Production: Algae can be used to produce various biofuels, including biodiesel, bioethanol, and biogas.
- Waste Water Treatment: Algae can be used in waste water treatment by absorbing nutrients.
- Disadvantages:
- High Production Costs: The current costs associated with algae cultivation and biofuel extraction are relatively high compared to fossil fuels.
- Scalability Challenges: Scaling up algae production to meet significant biofuel demands poses technological and logistical hurdles.
- Energy Input Requirements: Algae cultivation requires energy for various processes, including mixing, lighting, and harvesting, which can impact the overall energy balance.
- Water Consumption: While algae can be grown in saltwater, large-scale cultivation can still require significant water resources.
- Species Selection and Optimization: Finding and optimizing algae strains with high lipid content and efficient biofuel conversion processes is a complex task.
Step-by-Step Procedure for Converting Algae into Biofuel
The process of converting algae into biofuel involves several key steps, from cultivation to fuel production. The specific methods and technologies employed can vary depending on the type of biofuel being produced and the algae species used.
- Algae Cultivation: The process starts with the cultivation of algae. This involves selecting a suitable algae strain and growing it in a controlled environment, such as open ponds, closed photobioreactors, or raceway ponds. The environment must provide essential elements for algae growth, including sunlight, nutrients, and carbon dioxide.
- Harvesting: Once the algae have reached a sufficient biomass, they must be harvested. The harvesting method depends on the algae species and the cultivation system. Common methods include:
- Sedimentation: Allowing the algae to settle at the bottom of the cultivation system.
- Filtration: Using filters to remove algae from the water.
- Centrifugation: Spinning the algae mixture at high speeds to separate the algae cells.
- Drying: After harvesting, the algae biomass typically contains a high water content. Drying the algae is necessary to reduce the water content and increase the concentration of lipids and other useful compounds. Drying can be done using various methods, including:
- Sun Drying: The simplest method, but weather-dependent.
- Oven Drying: Provides more control over the drying process.
- Spray Drying: A more efficient method, especially for large-scale production.
- Lipid Extraction: The next step is extracting the lipids (oils) from the dried algae biomass. Several methods are available for lipid extraction:
- Solvent Extraction: Using solvents, such as hexane or ethanol, to dissolve the lipids.
- Mechanical Pressing: Using mechanical presses to squeeze the lipids out of the algae cells.
- Supercritical Fluid Extraction: Using supercritical fluids, such as carbon dioxide, to extract the lipids.
- Transesterification (for Biodiesel Production): If biodiesel is the desired end product, the extracted lipids undergo transesterification. This chemical process involves reacting the lipids with an alcohol (such as methanol or ethanol) in the presence of a catalyst (such as sodium hydroxide or potassium hydroxide) to produce fatty acid methyl esters (FAME), which is biodiesel.
Lipids + Alcohol → Biodiesel + Glycerol
- Other Biofuel Production Methods: Besides biodiesel, algae can be converted into other biofuels:
- Bioethanol: Algae biomass can be broken down into sugars, which are then fermented to produce bioethanol.
- Biogas: Algae biomass can be anaerobically digested to produce biogas, a mixture of methane and carbon dioxide.
- Fuel Refining and Purification: The resulting biofuel undergoes refining and purification to remove impurities and improve its quality. This may involve distillation, filtration, and other processes.
- Fuel Storage and Distribution: Finally, the refined biofuel is stored and distributed for use as a transportation fuel.
Illustrations/Images of Algae and Food Chains
Visual representations are critical to understanding the complex interactions within algal food chains and the environmental impact of algal activity. They offer a clear and concise way to convey information about species, energy flow, and the effects of imbalances in these ecosystems. The following sections describe detailed illustrations and images related to these concepts.
Algal Food Chain in a Freshwater Ecosystem
An illustration depicts a vibrant freshwater ecosystem, showcasing a simplified algal food chain. The illustration is dominated by a clear blue lake, reflecting the sky. At the base of the food chain, microscopic green algae, specifically
- Chlamydomonas* and
- Volvox*, are abundant, forming a dense, green cloud suspended in the water. These are depicted as small, spherical or ovoid structures, with internal details hinting at their cellular structure.
Above the algae, a group of primary consumers, the herbivores, are represented. These include small, transparent
- Daphnia* (water fleas), shown actively feeding on the algae. They are drawn with their characteristic two-part bodies, feathery antennae, and a visible digestive tract filled with green algae. Alongside the
- Daphnia*, larger organisms, like
- Paramecium*, are shown grazing on algae, representing a different feeding strategy.
Moving up the food chain, small carnivorous fish, such as
- Gambusia* (mosquito fish), are illustrated. These fish are depicted with streamlined bodies, actively pursuing the
- Daphnia* and other smaller invertebrates. The fish are shown with visible eyes and open mouths, capturing their prey.
Finally, at the apex of this simplified food chain, a larger predator, a Great Blue Heron, is perched on a rock at the water’s edge. It’s shown with its long legs, sharp beak, and watchful eye, ready to capture the fish. The illustration employs a clear and straightforward style, using labeled arrows to show the direction of energy flow from the algae to the herbivores, then to the carnivores, and finally to the top predator.
The background includes submerged aquatic plants, adding context to the ecosystem. The overall effect is a visually engaging and informative representation of the energy transfer within the freshwater food chain.
Impact of an Algal Bloom on a Coastal Environment
The image portrays a coastal environment devastated by an algal bloom, visually emphasizing the detrimental effects. The dominant feature is a large expanse of water, colored a murky reddish-brown. This discoloration represents the excessive growth of harmful algal species, likely
Karenia brevis*, which causes red tides.
Along the shoreline, numerous dead fish are depicted, floating on the surface or washed ashore. These fish are of various sizes and species, providing visual evidence of the impact of the bloom on the marine fauna. Their bodies show signs of decay, with open mouths and visible gills.The image also includes visual cues of the environmental consequences. Foam and froth are visible along the shoreline, indicating the decomposition of the algae and the release of toxins.
Seagrass beds are shown as being stressed and dying, their vibrant green color fading to brown, representing the impact on the benthic habitat.In the background, a hazy atmosphere suggests the presence of airborne toxins produced by the algal bloom, potentially affecting human health. The sky is overcast, adding to the overall sense of devastation.The overall effect is a powerful visual warning, illustrating the destructive potential of algal blooms and the far-reaching consequences for marine ecosystems and coastal communities.
The image is a stark reminder of the importance of monitoring and mitigating the causes of these harmful events.
Energy Flow within an Algal-Based Food Web
The diagram illustrates the energy flow within an algal-based food web, presenting the concept of trophic levels and energy transfer. The diagram uses a series of interconnected boxes and arrows to represent the flow of energy.At the base of the diagram, the primary producers, the algae, are represented by a large box. The algae are labeled with their role as the foundation of the food web, highlighting their photosynthetic ability to convert sunlight into energy.Above the algae, a series of boxes represent the different trophic levels.
These include primary consumers (herbivores), secondary consumers (carnivores), and tertiary consumers (top predators). Each level is clearly labeled, with examples of organisms found in each. For example, the primary consumer box contains organisms such as copepods and small crustaceans, while the secondary consumer box includes small fish.Arrows indicate the direction of energy flow, starting from the algae and moving up the food web.
The thickness of the arrows is used to represent the amount of energy transferred between each trophic level. The arrows become progressively thinner as they move up the food web, reflecting the decrease in energy availability at each successive level due to energy loss through respiration, waste, and other metabolic processes.The diagram also incorporates the concept of energy loss. The boxes for each trophic level are shaded to represent energy losses through respiration, waste, and heat.
The diagram also shows the decomposition of dead organisms and waste, returning nutrients to the algae, completing the cycle. The diagram is simple and clear, emphasizing the transfer of energy from the algae, the primary producers, up the food chain to the consumers. The diagram is designed to illustrate how energy flows through the food web, highlighting the role of each organism in the process.
Last Recap
In conclusion, food chain algae are far more than just simple aquatic plants; they are the cornerstone of ecological balance and the lifeblood of countless ecosystems. From their photosynthetic efficiency to their role in biofuel production, their significance is undeniable. Understanding the complexities of algae, their interactions within food webs, and the factors affecting their populations is crucial for managing and protecting aquatic environments.
By appreciating the critical role of these primary producers, we can strive towards a more sustainable and harmonious relationship with the natural world.