Food ingredient analysis is not merely a scientific process; it’s a crucial endeavor that safeguards public health and ensures the integrity of our food supply. This intricate examination, which explores the composition of every edible item, is more than just a label-reading exercise. It’s a deep dive into the building blocks of our meals, revealing the hidden complexities and potential risks lurking within.
Understanding the “what” and “how” of ingredient analysis is paramount for informed consumer choices and a robust food industry.
The primary objective of food ingredient analysis is to determine the precise composition of food products, from the macro-level (proteins, fats, carbohydrates) to the micro-level (vitamins, minerals, additives). This process employs a multifaceted approach, combining physical, chemical, and biological techniques. These methods, along with adherence to regulatory standards set by organizations like the FDA and EFSA, ensure that what we eat is safe, accurately labeled, and meets quality standards.
The ultimate goal is to protect consumers from adulterated or contaminated products, ensuring they receive the nutritional value promised on the packaging.
Introduction to Component Examination
Food is fundamental to life, and understanding its composition is crucial for health, safety, and informed decision-making. Evaluating what constitutes our edibles allows us to make informed choices, protect ourselves from potential hazards, and appreciate the complex science behind what we consume. This knowledge empowers us to maintain well-being and navigate the intricate world of food with confidence.Ingredient examination is the systematic process of identifying and analyzing the individual components of a food product.
It involves breaking down a food item to understand its constituent parts, their properties, and their potential impact. This process goes beyond simply listing ingredients; it delves into the details of what makes up a food.
Primary Goals of Ingredient Examination
The primary goals of ingredient examination are multifaceted and serve various purposes within the food industry and for consumers. They are vital for ensuring food safety, maintaining product quality, and providing accurate information.
- Ensuring Food Safety: Examining ingredients helps identify potential allergens, contaminants, and harmful substances. This includes detecting the presence of undeclared ingredients that could pose risks to specific populations, such as those with allergies. For example, thorough ingredient analysis can reveal the presence of gluten in a product labeled “gluten-free” or the inclusion of peanuts in a snack food. This helps prevent adverse reactions and illnesses.
- Maintaining Product Quality: Ingredient examination aids in assessing the quality of raw materials and finished products. By analyzing the components, manufacturers can ensure consistency in taste, texture, and nutritional value. For instance, in the production of coffee, the analysis of the bean’s chemical composition determines its aroma and flavor. The analysis of the fat content in chocolate is critical for achieving the desired texture.
- Providing Accurate Labeling Information: Ingredient examination is essential for providing consumers with accurate and transparent information about the food they purchase. This includes identifying all ingredients, their quantities, and nutritional information. Precise labeling is critical for consumers to make informed choices, especially for those with dietary restrictions or specific health concerns. The “Nutrition Facts” label on food packaging is a direct result of ingredient analysis.
- Detecting Adulteration and Fraud: Ingredient examination helps to identify instances of food fraud, such as the substitution of cheaper ingredients for more expensive ones. For example, the analysis of olive oil can detect the presence of cheaper oils like sunflower or canola oil. This practice is deceptive and can mislead consumers.
- Optimizing Product Development: Ingredient examination plays a crucial role in product development. By understanding the properties of different ingredients, food scientists can create new products with desired characteristics, such as improved taste, shelf life, and nutritional value.
Methods for Evaluating Edible Components
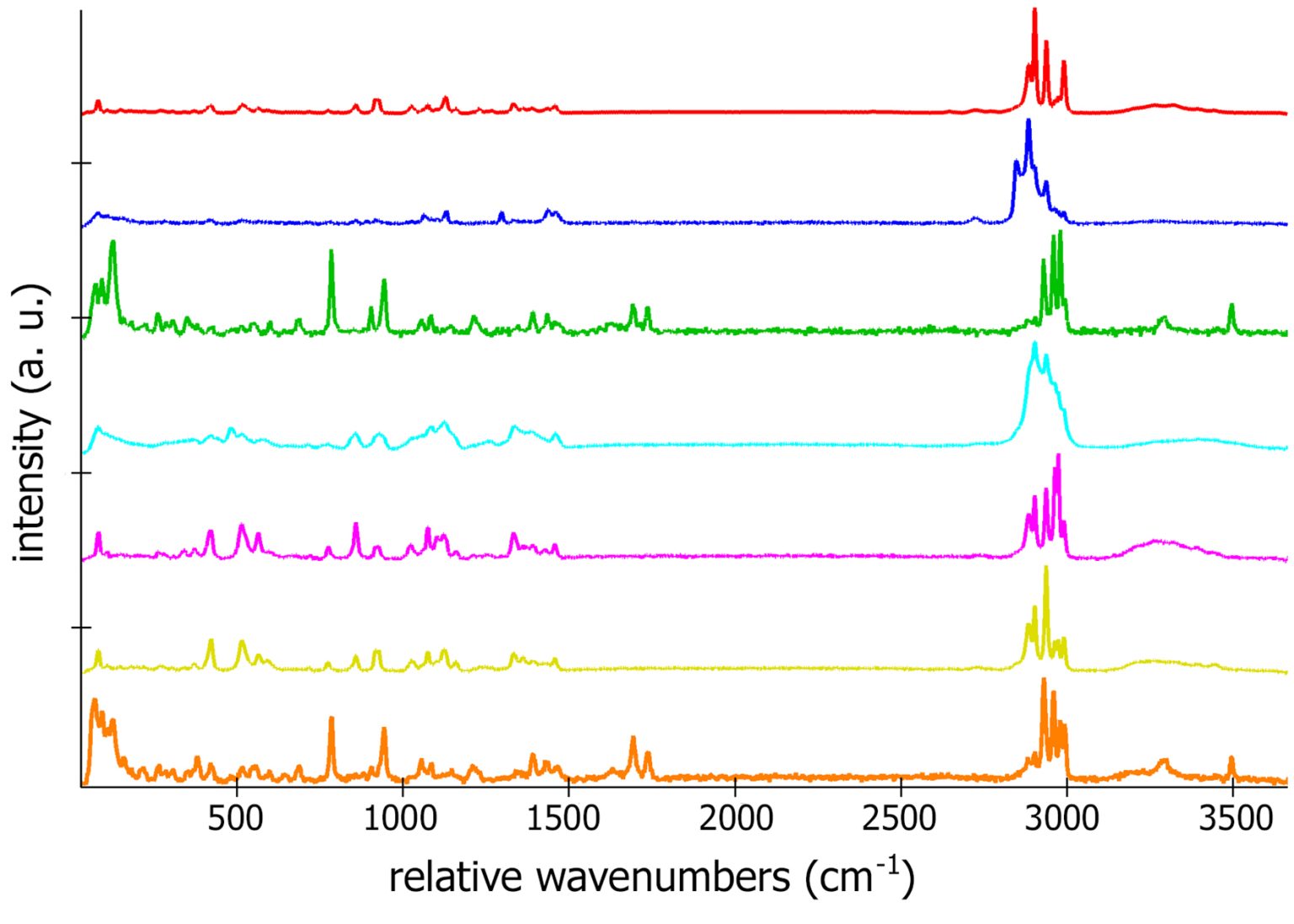
Hello there! Now that we’ve covered the basics of component examination, let’s delve into the exciting world ofhow* we actually evaluate the edible components within our food. Understanding the methods used is crucial for ensuring food safety, quality, and nutritional value. We’ll explore the different techniques scientists and food professionals employ to scrutinize what we eat.
Physical Methods for Component Examination
Physical methods are often the first line of investigation, providing quick and non-destructive ways to assess food components. These techniques measure properties like size, shape, color, and texture. They are usually straightforward to perform and can reveal a lot about the overall quality of a food product.
- Microscopy: Microscopy, using both light and electron microscopes, is used to visualize the microscopic structure of food components. This allows for the identification of cells, tissues, and particles, as well as the detection of contaminants. For example, microscopy can be used to examine the structure of starch granules in different flours or to identify the presence of microorganisms. A scanning electron microscope (SEM) provides high-resolution, three-dimensional images of food surfaces, revealing details about texture and structure at the nanoscale.
- Spectrophotometry: This technique measures the absorbance or transmission of light through a sample. It is widely used to determine the color and clarity of food products, as well as to quantify the concentration of specific components, such as pigments (e.g., carotenoids in carrots) or preservatives.
- Rheology: Rheological methods assess the flow and deformation properties of food materials. This includes viscosity, elasticity, and plasticity, which are crucial for understanding the texture and mouthfeel of foods. Instruments like viscometers and texture analyzers are used to measure these properties. For example, rheology is used to determine the thickness of sauces, the firmness of fruits, or the chewiness of bread.
- Chromatography: While often used in conjunction with chemical analysis, some chromatographic techniques, such as size exclusion chromatography (SEC), can be considered physical methods. SEC separates molecules based on their size, allowing for the determination of molecular weight distributions of components like proteins or polysaccharides.
- Sensory Evaluation: Though subjective, sensory evaluation by trained panelists is a crucial physical method. It assesses the appearance, aroma, taste, and texture of food products. This provides valuable information about consumer acceptance and can be used to identify changes in product quality over time. For example, a trained panel might evaluate the crispness of potato chips or the sweetness of a beverage.
Advantages: Physical methods are often non-destructive, fast, and relatively inexpensive. They can provide valuable information about the overall quality and characteristics of food products. They are also suitable for quality control and routine analysis.
Disadvantages: Physical methods alone may not provide detailed information about the chemical composition of food. They can be subjective (e.g., sensory evaluation) and may be influenced by factors such as the sample preparation or the operator’s skill.
Chemical Methods for Component Examination
Chemical methods provide detailed information about the composition of food, identifying and quantifying the different chemical compounds present. These methods are essential for determining nutritional content, detecting contaminants, and ensuring food safety. They often involve breaking down the food sample and using chemical reactions to analyze its components.
- Titration: Titration is a classical chemical technique used to determine the concentration of a substance by reacting it with a solution of known concentration (the titrant). It is commonly used to determine the acidity of foods (e.g., measuring the amount of acid in vinegar) or the concentration of preservatives.
- Spectroscopy: Various spectroscopic techniques are employed to analyze the interaction of light with food components.
- Infrared Spectroscopy (IR): Identifies functional groups within molecules, providing information about the chemical composition of the food.
- Ultraviolet-Visible Spectroscopy (UV-Vis): Measures the absorption of ultraviolet and visible light to quantify specific compounds, such as vitamins or food colorants.
- Chromatography: Chromatography separates and identifies individual components within a complex mixture. Different types of chromatography are used, including:
- Gas Chromatography (GC): Separates volatile compounds, often used to analyze flavor compounds, pesticides, or fatty acids.
- High-Performance Liquid Chromatography (HPLC): Separates non-volatile compounds, used for analyzing vitamins, amino acids, and sugars.
- Thin-Layer Chromatography (TLC): A simple and rapid technique used for separating and identifying compounds based on their polarity.
- Mass Spectrometry (MS): MS measures the mass-to-charge ratio of ions, providing highly accurate information about the molecular weight and structure of food components. It is often used in conjunction with chromatography (GC-MS, LC-MS) to identify and quantify complex mixtures of compounds.
- Enzyme-Linked Immunosorbent Assay (ELISA): ELISA is a biochemical technique used to detect and quantify the presence of specific proteins, such as allergens or toxins, in food samples. It uses antibodies to bind to the target molecules.
Advantages: Chemical methods provide detailed and quantitative information about the composition of food. They are highly specific and sensitive, allowing for the detection of even trace amounts of components. They are crucial for ensuring food safety and quality control.
Disadvantages: Chemical methods can be time-consuming and require specialized equipment and trained personnel. They may involve the use of hazardous chemicals and can be more expensive than physical methods.
Biological Methods for Component Examination
Biological methods involve using living organisms or their components to analyze food components. These methods are particularly important for assessing food safety and detecting the presence of microorganisms or toxins.
- Microbiological Analysis: This involves culturing food samples on specific growth media to identify and quantify the presence of microorganisms, such as bacteria, yeasts, and molds. Techniques include:
- Plate Counts: Enumerating the number of viable microorganisms in a sample.
- Most Probable Number (MPN): Estimating the number of microorganisms based on the growth in a series of dilutions.
- Molecular Biology Techniques: Such as Polymerase Chain Reaction (PCR), used to detect and identify specific microorganisms based on their genetic material.
- Bioassays: Bioassays use living organisms (e.g., animals, cells) to detect and quantify the presence of specific substances or their effects. They can be used to assess the toxicity of food components or to determine the biological activity of vitamins or other nutrients.
- Immunological Assays: Immunological assays, like ELISA, can be considered biological methods. They utilize antibodies to detect and quantify the presence of specific proteins, such as allergens or toxins.
Advantages: Biological methods are essential for assessing food safety and detecting the presence of harmful microorganisms or toxins. They can provide information about the biological activity and potential health effects of food components.
Disadvantages: Biological methods can be time-consuming and require specialized equipment and expertise. They may be affected by environmental factors and can be less precise than chemical methods.
Common Laboratory Equipment Used in Component Evaluation
A variety of laboratory equipment is used in food component analysis, depending on the specific methods employed. Here are some examples:
- Balances: Used for accurately weighing food samples and reagents.
- Microscopes: Used for visualizing the microscopic structure of food components.
- Spectrophotometers: Used for measuring the absorbance or transmission of light through a sample.
- Chromatographs (GC, HPLC): Used for separating and identifying individual components.
- Mass Spectrometers (MS): Used for determining the mass-to-charge ratio of ions.
- pH meters: Used for measuring the acidity or alkalinity of food samples.
- Viscometers and Texture Analyzers: Used for measuring the flow and deformation properties of food materials.
- Incubators and Autoclaves: Used for culturing microorganisms and sterilizing equipment.
- Centrifuges: Used for separating components based on density.
- Extraction Equipment: Soxhlet extractors, ultrasonic baths, etc. are used to isolate specific compounds from food matrices.
Procedures for a Simple Component Test: Starch Detection
Here’s a bullet-point list of procedures for a simple starch detection test using iodine solution:
- Sample Preparation: Obtain a small sample of the food material to be tested (e.g., a piece of bread, potato, or flour).
- Iodine Solution Preparation: Prepare a dilute iodine solution (e.g., 0.1% iodine in water or a potassium iodide solution).
-Warning: Iodine can stain and is a mild irritant; handle with care.* - Sample Application: Place a small amount of the food sample on a clean, white surface (e.g., a white ceramic tile or a white plate).
- Iodine Application: Add a few drops of the iodine solution to the food sample.
- Observation: Observe the sample for any color change.
- Interpretation:
- Blue-Black Color: Indicates the presence of starch. The iodine reacts with the amylose component of starch to form a blue-black complex.
- No Color Change or a Yellowish-Brown Color: Indicates the absence of starch or a very low concentration.
Physical Examination Techniques
Hello food enthusiasts! Now that we’ve explored the foundational aspects of food component analysis, let’s delve into the fascinating world of physical examination techniques. These techniques offer a non-destructive and often straightforward approach to understanding the characteristics of food ingredients. They’re the first line of defense in assessing quality and identifying potential issues. This section will explore the various methods employed, their applications across different food types, and how they can be used to detect ingredient adulteration.
Visual Inspection
Visual inspection is a fundamental technique that relies on the sense of sight to assess the physical attributes of a food item. It’s often the initial step in food analysis and can provide valuable insights into quality, freshness, and potential issues.
- Color: Color can be a significant indicator of freshness, processing methods, and the presence of certain compounds. For example, the browning of apples can indicate oxidation, while the vibrant red of tomatoes signifies ripeness and the presence of lycopene. Variations in color can also signal the presence of additives or adulterants.
- Shape and Size: The shape and size of food items can be critical for assessing quality and detecting deviations from expected norms. For instance, the uniform size of pasta shapes indicates proper manufacturing, while misshapen or broken pieces might suggest poor quality. The size of fruit can indicate ripeness or variety.
- Appearance of Surface: The surface characteristics, such as smoothness, glossiness, and the presence of defects, are important. A glossy surface might indicate the presence of fats or oils, while a rough or pitted surface could suggest spoilage or damage. The presence of mold or insects is also easily detected through visual inspection.
- Presence of Foreign Materials: Visual inspection is crucial for identifying foreign materials that may have contaminated the food product during processing or packaging. This includes items like hair, insects, pieces of packaging, or other non-food components.
Texture Analysis
Texture analysis evaluates the physical properties of food that are perceived through touch. It involves assessing characteristics like firmness, chewiness, viscosity, and mouthfeel. Texture analysis is crucial for determining the overall quality and consumer acceptance of food products.
- Firmness: Firmness is the resistance of a food product to deformation. It is a critical characteristic in many foods, such as fruits and vegetables.
- Chewiness: Chewiness is the length of time required to chew a food item before swallowing. It’s often associated with the fibrous nature of the food.
- Viscosity: Viscosity is the resistance of a fluid to flow. It’s particularly important in liquids like sauces, soups, and beverages.
- Mouthfeel: Mouthfeel describes the overall sensory experience in the mouth, including attributes such as smoothness, grittiness, and stickiness.
Texture analysis is often performed using specialized instruments like texture analyzers, which measure the force, distance, and time required to deform a food sample. These instruments can provide objective and quantifiable data on textural properties. For example, the texture of bread can be assessed by measuring its firmness using a texture analyzer. The results will provide data about the force required to compress a bread sample, which helps in assessing its freshness.
Density Measurements
Density is a fundamental physical property defined as mass per unit volume. Measuring the density of food ingredients provides valuable information about their composition, purity, and potential adulteration.
- Importance of Density: Density measurements are particularly useful in identifying adulteration. For instance, adding water to honey will decrease its density, and adding sugar to milk will increase its density.
- Methods of Measurement: Density can be determined using various methods, including the pycnometer method, the hydrometer method, and the buoyancy method.
- Pycnometer Method: This method involves weighing a known volume of the food sample using a pycnometer (a small glass flask with a precise volume). The density is then calculated using the formula:
Density = Mass / Volume
- Hydrometer Method: A hydrometer is a glass instrument that sinks in a liquid until the weight of the liquid it displaces equals the weight of the hydrometer. The hydrometer is calibrated to read the density of the liquid directly.
Identifying Ingredient Adulteration Through Physical Examination
Physical examination techniques play a critical role in detecting ingredient adulteration, which is the practice of adding inferior substances to food products to increase profits or deceive consumers.
- Visual Inspection Examples:
- Olive Oil: Visual inspection can reveal the presence of other oils. For example, a lighter color or a less viscous appearance might suggest the addition of cheaper vegetable oils.
- Honey: The presence of crystallized sugar or a cloudy appearance can indicate adulteration with sugar syrups.
- Texture Analysis Examples:
- Flour: Texture analysis can detect the addition of starch or other fillers to flour, which would alter its firmness and cohesiveness.
- Ground Meat: A change in the texture of ground meat, such as increased stickiness or a less firm consistency, could indicate the addition of water or other binding agents.
- Density Measurement Examples:
- Milk: A decrease in the density of milk might suggest the addition of water.
- Fruit Juices: The density of fruit juice can be used to detect the addition of water or sugar syrups.
Experiment: Measuring the Density of a Common Food Item (Honey)
This simple experiment demonstrates how to measure the density of honey using the pycnometer method.
- Materials:
- Pycnometer (or a small, calibrated flask with a known volume)
- Analytical balance
- Honey sample
- Distilled water (for calibration)
- Procedure:
- Calibration: Weigh the empty pycnometer. Fill the pycnometer with distilled water. Weigh the pycnometer filled with water. Calculate the volume of the pycnometer using the density of water (approximately 1 g/mL at room temperature):
Volume of pycnometer = (Weight of pycnometer + water – Weight of empty pycnometer) / Density of water
- Honey Sample: Clean and dry the pycnometer. Weigh the empty pycnometer. Fill the pycnometer with honey. Weigh the pycnometer filled with honey.
- Calculation: Calculate the density of honey:
Density of honey = (Weight of pycnometer + honey – Weight of empty pycnometer) / Volume of pycnometer
- Calibration: Weigh the empty pycnometer. Fill the pycnometer with distilled water. Weigh the pycnometer filled with water. Calculate the volume of the pycnometer using the density of water (approximately 1 g/mL at room temperature):
- Expected Results: The density of honey typically ranges from 1.36 to 1.44 g/mL, depending on the floral source and water content. If the measured density is significantly lower, it might suggest adulteration with water or other substances.
Chemical Examination Techniques
Welcome back! Having explored physical examination techniques, we now turn our attention to the fascinating world of chemical examination. This realm delves deep into the molecular composition of food ingredients, offering a powerful toolkit for understanding their quality, safety, and nutritional value. Chemical analysis provides a microscopic view, revealing the intricate details that often remain hidden from the naked eye.
Role of Chemical Examination
Chemical examination plays a crucial role in food ingredient analysis by providing detailed information about the presence and quantity of various components. This includes identifying the building blocks of food, such as proteins, carbohydrates, and fats, as well as detecting additives, preservatives, and potential contaminants. The techniques employed allow for a thorough assessment of a food ingredient’s chemical makeup, enabling the determination of its authenticity, safety, and overall quality.
Chromatography, spectroscopy, and titrations are among the core methodologies utilized in this process.
Chromatography
Chromatography is a separation technique that separates the components of a mixture based on their different physical and chemical properties. This method is essential for isolating and identifying individual compounds within a complex food matrix.
- Principles: Chromatography works by passing a sample through a stationary phase (a solid or a liquid supported on a solid) and a mobile phase (a liquid or a gas). The different components of the sample interact differently with the stationary and mobile phases, causing them to travel at different rates and separate from each other.
- Types of Chromatography: Several types of chromatography are used in food analysis, including:
- Gas Chromatography (GC): Used to separate volatile compounds, such as flavors and aromas.
- High-Performance Liquid Chromatography (HPLC): Used to separate non-volatile compounds, such as vitamins, sugars, and amino acids.
- Thin-Layer Chromatography (TLC): A simple and cost-effective method used for the initial separation and identification of compounds.
- Applications: Chromatography is used to determine the presence and quantity of various components, such as:
- Fatty acid profiles: Determining the type and amount of different fatty acids in oils and fats.
- Sugar analysis: Quantifying the levels of different sugars in foods.
- Pesticide residue detection: Identifying and quantifying pesticide residues in fruits and vegetables.
Spectroscopy
Spectroscopy utilizes the interaction of electromagnetic radiation with matter to identify and quantify substances. It’s a powerful tool for revealing the molecular structure and composition of food ingredients.
- Principles: Spectroscopy works by measuring how a substance absorbs, emits, or scatters light (or other forms of electromagnetic radiation) at different wavelengths. The unique spectral “fingerprint” of a substance can then be used to identify and quantify it.
- Types of Spectroscopy: Several types of spectroscopy are used in food analysis, including:
- Ultraviolet-Visible (UV-Vis) Spectroscopy: Used to measure the absorption of light in the ultraviolet and visible regions of the electromagnetic spectrum. This technique is used to determine the concentration of colored compounds in foods, such as pigments and dyes.
- Infrared (IR) Spectroscopy: Used to identify and quantify organic molecules by measuring the absorption of infrared radiation. This is helpful in determining the presence of specific functional groups (e.g., carbonyl groups in fats or hydroxyl groups in carbohydrates) and identifying adulteration.
- Mass Spectrometry (MS): A technique used to measure the mass-to-charge ratio of ions. It is often coupled with chromatography (GC-MS or LC-MS) to identify and quantify individual components in a complex mixture.
- Applications: Spectroscopy is used for a variety of purposes, including:
- Authenticity testing: Identifying the presence of adulterants or contaminants. For instance, UV-Vis spectroscopy can be used to detect the addition of artificial dyes to fruit juices.
- Nutritional analysis: Determining the levels of specific nutrients, such as vitamins or proteins.
- Quality control: Assessing the quality of food products by measuring the levels of specific compounds, such as antioxidants or preservatives.
Titrations
Titration is a quantitative chemical analysis technique used to determine the concentration of a substance (the analyte) by reacting it with a solution of known concentration (the titrant). This method provides a simple and accurate way to measure the amount of a specific component in a food sample.
- Principles: Titration involves gradually adding a titrant to a solution of the analyte until the reaction is complete, as indicated by a change in color (using an indicator), or a change in electrical conductivity. The volume of titrant used is then used to calculate the concentration of the analyte.
- Types of Titrations:
- Acid-Base Titration: Used to determine the acidity or basicity of a substance.
- Redox Titration: Used to determine the concentration of a substance that can be oxidized or reduced.
- Complexometric Titration: Used to determine the concentration of metal ions.
- Applications: Titration is used in food analysis for:
- Measuring acidity: Determining the acidity of fruit juices, wines, and other acidic foods. For example, titratable acidity in orange juice can be measured to assess its freshness and quality.
- Determining salt content: Assessing the salt content of processed foods.
- Measuring the concentration of preservatives: Quantifying the amount of preservatives, such as sodium benzoate or potassium sorbate, in food products.
Examples of Chemical Tests
Numerous chemical tests are employed to detect additives, preservatives, and contaminants in food. These tests are essential for ensuring food safety and quality.
- Detection of Additives: Tests can identify the presence and concentration of artificial colors, flavor enhancers (such as MSG), and artificial sweeteners. For instance, a colorimetric test can be used to detect the presence of tartrazine (a yellow dye) in beverages.
- Detection of Preservatives: Tests can identify the presence and concentration of preservatives such as benzoates, sorbates, and sulfites. For example, a spectrophotometric method can quantify the amount of sulfur dioxide (a sulfite preservative) in dried fruits.
- Detection of Contaminants: Tests can detect the presence of contaminants, such as heavy metals (lead, mercury), pesticide residues, and mycotoxins (produced by molds). For example, an ELISA (enzyme-linked immunosorbent assay) can detect the presence of aflatoxins (a type of mycotoxin) in grains and nuts.
Common Chemical Tests and Their Measurements
The following table summarizes common chemical tests and what they measure in food ingredient analysis:
Chemical Test | Technique | Measured Component | Example Application |
---|---|---|---|
pH Measurement | Electrometry | Acidity/Alkalinity | Determining the acidity of fruit juices or wines. |
Titration (Acid-Base) | Volumetric Analysis | Titratable Acidity | Measuring the acidity of vinegar or the amount of acidulants in processed foods. |
HPLC | Chromatography | Sugars, Vitamins, Amino Acids | Quantifying the sugar content in honey or measuring vitamin C levels in orange juice. |
GC-MS | Chromatography & Mass Spectrometry | Volatile Compounds, Pesticide Residues | Analyzing the flavor profile of coffee or detecting pesticide residues in fruits and vegetables. |
UV-Vis Spectroscopy | Spectroscopy | Colorants, Preservatives | Detecting artificial dyes in beverages or quantifying the concentration of preservatives in processed foods. |
IR Spectroscopy | Spectroscopy | Functional Groups, Adulterants | Identifying the type of fat in cooking oil or detecting adulteration in olive oil. |
ELISA | Immunological Assay | Allergens, Mycotoxins | Detecting allergens in food products or identifying the presence of mycotoxins in grains. |
Biological Examination Techniques: Food Ingredient Analysis
Welcome back to our exploration of food ingredient analysis! We’ve covered a lot of ground, from understanding components to various examination methods. Now, let’s delve into the fascinating world of biological examination techniques. These methods are crucial for ensuring the safety and quality of the food we consume, focusing on the living organisms and their impact on our edibles. This segment will explore how we utilize these techniques to detect and assess the presence of microorganisms and other biological elements that could affect food safety.
Application of Biological Methods
Biological methods are essential tools in food analysis, offering a unique perspective on the composition and safety of ingredients. These methods primarily focus on identifying and quantifying biological entities, such as microorganisms and allergens, which can significantly impact food quality and consumer health. The application of these methods is diverse, ranging from routine quality control checks to investigations of foodborne illness outbreaks.Microbial testing is a cornerstone of food safety, involving the detection and quantification of microorganisms like bacteria, yeasts, and molds.
This testing helps determine if a food product meets the required safety standards and is free from harmful pathogens. Allergen detection, another critical application, identifies the presence of specific proteins that can trigger allergic reactions in sensitive individuals. This is crucial for proper labeling and ensuring consumer safety. The application of these methods ensures the food products meet stringent safety standards, safeguarding public health.
Examples of Methods for Ensuring Safety and Quality
Biological methods play a vital role in guaranteeing the safety and quality of food products. Here are some practical examples of how these methods are employed in the food industry:* Microbial Testing in Dairy Products: Milk and dairy products are frequently tested for the presence of
- Salmonella*,
- Listeria monocytogenes*, and
- E. coli*. The detection of these pathogens can indicate potential contamination during processing or storage, necessitating immediate corrective actions.
Allergen Detection in Processed Foods
Many processed foods undergo rigorous allergen testing to verify the absence of common allergens, such as peanuts, soy, gluten, and milk. Techniques like ELISA (Enzyme-Linked Immunosorbent Assay) are employed to detect even trace amounts of allergenic proteins, ensuring accurate labeling and consumer safety.
Yeast and Mold Counts in Baked Goods
Bakery products are tested to ensure that they are free from excessive levels of yeast and mold. These organisms can cause spoilage, affecting the product’s appearance, texture, and flavor. This testing helps maintain the product’s shelf life and overall quality.
These examples showcase how biological techniques are integrated into food processing and quality control procedures to protect consumers from potential health hazards and maintain product integrity.
Comparison of Different Biological Tests for Specific Components
Various biological tests are available for assessing different components in food. The choice of test depends on the target analyte (the substance being measured), the desired sensitivity, and the specific requirements of the analysis. Here’s a comparison of some commonly used tests:* Microbial Tests:
Culture-based methods
These involve growing microorganisms in a suitable medium and then identifying them based on their characteristics. They are highly specific but can be time-consuming, typically taking several days to produce results.
Polymerase Chain Reaction (PCR)
PCR is a molecular technique that amplifies specific DNA sequences. It offers high sensitivity and speed, allowing for rapid detection of pathogens. However, it requires specialized equipment and expertise.
Immunoassay-based methods (e.g., ELISA)
These tests use antibodies to detect specific microbial antigens. They are relatively quick and can be used for various pathogens, but their sensitivity may vary.
Allergen Detection Tests
ELISA (Enzyme-Linked Immunosorbent Assay)
This is a widely used method that uses antibodies to detect and quantify allergen proteins. It offers high sensitivity and is relatively easy to perform.
Lateral flow devices
These are rapid, portable tests that provide quick results. They are commonly used for on-site allergen detection but may have lower sensitivity compared to ELISA.
PCR-based methods
These methods can detect allergen DNA, providing a sensitive and specific analysis.The selection of the appropriate test involves balancing factors such as speed, sensitivity, cost, and the specific requirements of the food analysis. For instance, a rapid test might be suitable for on-site screening, while a more sensitive method is required for accurate quantification in a laboratory setting.
Steps in a Microbial Test for a Common Foodborne Pathogen
Microbial tests typically follow a standardized process to ensure accuracy and reliability. Here’s a general Artikel of the steps involved in a microbial test for a common foodborne pathogen, such as
Salmonella*
1. Sample Collection
A representative sample of the food product is collected and transferred to a sterile container. The sample size depends on the type of food and the testing requirements.
2. Pre-enrichment
The sample is added to a non-selective enrichment broth, such as buffered peptone water. This step allows anySalmonella* present to recover and multiply without competition from other organisms. The broth is incubated at a specific temperature for a specific period.
-
3. Selective Enrichment
After pre-enrichment, the sample is transferred to a selective enrichment broth, such as Rappaport-Vassiliadis broth. This broth contains selective agents that inhibit the growth of nonSalmonella* bacteria, allowing
- Salmonella* to grow.
- Salmonella* colonies from other bacteria.
- Salmonella* colonies to grow and become visible.
- Salmonella* colonies typically have specific morphological characteristics.
- Salmonella*. These tests include tests for specific enzyme production and sugar fermentation.
- Salmonella* serotype.
- Salmonella* was detected in the sample and, if so, the number of colony-forming units (CFU) per gram or milliliter of the food product.
4. Plating on Selective Agar
The enriched sample is then streaked onto a selective agar plate, such as Xylose Lysine Desoxycholate (XLD) agar or Hektoen Enteric (HE) agar. These agars contain selective agents and indicators that help differentiate
5. Incubation
The agar plates are incubated at a specific temperature (typically 37°C) for a specified time (usually 24-48 hours). This allows any
6. Colony Identification
Suspect colonies are examined for their characteristic appearance on the agar plates.
7. Biochemical Tests
Selected colonies are subjected to biochemical tests to confirm the presence of
8. Serological Confirmation
Serological tests, such as agglutination tests using specific antibodies, may be used to confirm the identity of the
9. Results and Reporting
The results of the test are recorded and reported. The report indicates whether
These steps ensure that the food product is thoroughly tested for the presence of
Salmonella* and other potential pathogens, helping to maintain food safety standards.
Regulatory Aspects and Standards
In the realm of food ingredient analysis, adherence to regulations and standards is not merely a suggestion; it’s a fundamental requirement. These guidelines safeguard public health, ensure fair market practices, and facilitate international trade. Understanding and complying with these regulatory frameworks is paramount for manufacturers, researchers, and consumers alike. This section will delve into the critical role of regulations and standards in component evaluations, the influence of key organizations, and practical examples of how these regulations manifest in food labeling.
Importance of Regulations and Standards in Component Evaluations
Regulations and standards play a crucial role in ensuring the safety, quality, and transparency of food products. They establish a framework for evaluating the components of food ingredients, covering aspects such as safety, purity, and nutritional value.
- Public Health Protection: Regulations set limits on harmful substances like pesticides, heavy metals, and microbial contaminants, protecting consumers from potential health risks.
- Quality Assurance: Standards define acceptable levels of components, ensuring consistent product quality and preventing adulteration. For instance, standards might specify the minimum protein content in a particular type of flour.
- Consumer Protection: Regulations mandate accurate labeling, providing consumers with essential information about ingredients, nutritional content, and potential allergens, enabling informed choices.
- Fair Trade Practices: Standardized testing methods and labeling requirements promote fair competition and prevent deceptive practices in the food industry.
- Facilitating International Trade: Harmonized standards between countries ease the process of importing and exporting food products, reducing trade barriers.
Influence of Organizations like the FDA and EFSA on Component Standards
Several organizations worldwide exert significant influence on component standards. The Food and Drug Administration (FDA) in the United States and the European Food Safety Authority (EFSA) in Europe are among the most prominent. These organizations develop and enforce regulations, conduct risk assessments, and provide scientific advice on food safety matters.
- Food and Drug Administration (FDA): The FDA is responsible for ensuring the safety and proper labeling of most food products in the US. It sets standards for food additives, contaminants, and nutritional labeling. The FDA’s regulations, such as those related to Generally Recognized as Safe (GRAS) substances, are critical in determining the acceptability of food components.
- European Food Safety Authority (EFSA): EFSA provides independent scientific advice on risks associated with the food chain in the European Union. It assesses the safety of food ingredients, including additives, novel foods, and genetically modified organisms (GMOs). EFSA’s opinions influence the European Commission’s decisions on food safety regulations. For example, EFSA’s evaluation of a food additive determines whether it can be used in the EU and under what conditions.
- International Collaboration: Both the FDA and EFSA often collaborate with other international organizations, such as the World Health Organization (WHO) and the Food and Agriculture Organization (FAO), to harmonize food safety standards and promote global food safety.
Examples of Labeling Requirements Based on Component Evaluations
Component evaluations directly influence the information displayed on food labels. These requirements aim to provide consumers with accurate and useful information to make informed decisions.
- Nutritional Information: Labels must include a nutrition facts panel detailing the amount of macronutrients (proteins, carbohydrates, fats), micronutrients (vitamins, minerals), and calories per serving. This information is derived from component analysis of the food.
- Ingredient List: All ingredients must be listed in descending order of weight. This ensures transparency about the composition of the food product. For instance, a cereal label will list the grains, sugars, and any additives used.
- Allergen Declarations: Labels must clearly identify common allergens, such as milk, eggs, peanuts, tree nuts, soy, wheat, and shellfish. This is a critical requirement for protecting individuals with food allergies.
- Additive Information: The presence of food additives, such as preservatives, colorings, and flavorings, must be declared on the label. This allows consumers to avoid specific additives if they choose.
- Health Claims: Any health claims made on the label, such as “low fat” or “good source of fiber,” must be substantiated by scientific evidence and meet specific criteria defined by regulatory agencies.
Comparison of Labeling Regulations for Different Regions
Labeling regulations vary significantly across different regions, reflecting diverse cultural preferences, scientific understanding, and regulatory priorities. The following table compares key aspects of labeling regulations in the US and the EU.
Feature | United States (FDA) | European Union (EFSA) |
---|---|---|
Nutrition Facts Panel | Requires a standardized format for displaying nutritional information, including serving size, calories, macronutrients, and micronutrients. The format has been updated to emphasize serving sizes and include added sugars. | Requires a similar nutrition declaration but allows for more flexibility in format. The declaration often includes the “Nutrition Declaration” or “Nutritional Information” section, presenting energy and nutrient information per 100g or 100ml, with optional per-serving information. |
Ingredient Listing | Ingredients are listed in descending order of weight. Common or usual names must be used for ingredients. | Ingredients are also listed in descending order of weight. The EU has stricter rules on the use of specific ingredient names and requires the declaration of certain ingredients, even in small quantities. |
Allergen Labeling | Requires the declaration of major food allergens in plain language, usually within the ingredient list or in a separate “Contains” statement. The “Food Allergen Labeling and Consumer Protection Act of 2004” mandated the labeling of the top eight allergens. | Requires the declaration of 14 specific allergens, with clear labeling. Allergens must be emphasized typographically within the ingredient list (e.g., bold, italicized, or a different font). |
Genetically Modified Organisms (GMOs) | Currently, the US does not require mandatory labeling of GMOs. However, the National Bioengineered Food Disclosure Standard (NBFDS) mandates the disclosure of bioengineered foods. | The EU has mandatory labeling requirements for GMOs. Food products containing more than 0.9% of genetically modified ingredients must be labeled as such. This is enforced to inform consumer choices. |
Nutritional Component Evaluations
Understanding the nutritional composition of food is crucial for both consumers and the food industry. Component examination plays a vital role in determining this composition, providing the data necessary for informed dietary choices, product development, and regulatory compliance. This section delves into how component examination is used to assess the nutritional content of foods, covering methods for evaluating vitamins, minerals, and macronutrients, informing nutritional labeling, and calculating nutritional values.
Determining Nutritional Content Through Component Examination
Component examination utilizes various analytical techniques to quantify the presence and amounts of nutrients within a food product. These techniques provide the foundation for understanding a food’s nutritional profile, which is essential for assessing its health benefits and potential risks. The data gathered through these methods are critical for making informed decisions about food consumption and for developing healthier food products.
Evaluating the Presence of Vitamins, Minerals, and Macronutrients, Food ingredient analysis
Evaluating the presence of essential nutrients involves employing specific analytical methods tailored to each nutrient type. These methods are designed to accurately measure the concentrations of vitamins, minerals, and macronutrients, providing a comprehensive understanding of the food’s nutritional value.
- Vitamins: Vitamin analysis often relies on chromatographic techniques, such as High-Performance Liquid Chromatography (HPLC), and mass spectrometry. These methods separate and identify individual vitamins within a food sample. For example, the concentration of vitamin C (ascorbic acid) can be determined using HPLC, where the vitamin is separated from other components and quantified based on its absorption of UV light.
- Minerals: Mineral analysis typically involves techniques like Atomic Absorption Spectrometry (AAS) or Inductively Coupled Plasma Atomic Emission Spectroscopy (ICP-AES). These methods measure the concentration of specific minerals by detecting the light emitted or absorbed by the mineral atoms when subjected to specific energy levels. For instance, the calcium content in milk can be determined using ICP-AES, providing accurate measurements of the mineral’s presence.
- Macronutrients: Macronutrient analysis focuses on carbohydrates, proteins, and fats.
- Carbohydrates: Carbohydrate analysis involves techniques like enzymatic methods to measure specific sugars (e.g., glucose, fructose) and chromatographic methods to determine the composition of dietary fiber.
- Proteins: Protein analysis often utilizes the Kjeldahl method to determine the total nitrogen content, which is then converted to protein content using a conversion factor. Amino acid analysis, employing chromatography, can also be used to determine the specific amino acid profile of a protein.
- Fats: Fat analysis involves extracting lipids from the food sample and then determining the fatty acid profile using gas chromatography. This reveals the types and amounts of saturated, monounsaturated, and polyunsaturated fatty acids present.
Informing Nutritional Labeling Through Component Examination
Component examination provides the data necessary for accurate and compliant nutritional labeling. This information is essential for consumers to make informed choices and for food manufacturers to meet regulatory requirements.
- Nutrient Content Claims: Component examination results are used to support nutrient content claims, such as “high in fiber” or “low in sodium.” These claims must be substantiated by analytical data demonstrating that the food product meets the specific criteria set by regulatory agencies.
- Ingredient Lists: The results from component examination also inform the ingredient list on a food label. For example, the presence and quantity of allergens must be clearly stated on the label, and this information is derived from component analysis.
- Nutritional Facts Panel: The nutritional facts panel, including serving size, calories, macronutrients, vitamins, and minerals, is directly based on the results of component examination. The data from these analyses are used to calculate the values displayed on the panel.
Calculating the Nutritional Value of a Food Product
Calculating the nutritional value of a food product involves using the results of component examination to determine the amount of each nutrient per serving. This process provides a comprehensive overview of the food’s nutritional profile, which is then presented in the nutritional facts panel.
- Determining Serving Size: The serving size is determined based on the typical amount of food consumed.
- Quantifying Nutrients Per Serving: The concentration of each nutrient is multiplied by the serving size to calculate the amount of that nutrient per serving.
- Energy Calculation: The energy content (calories) is calculated based on the amounts of macronutrients (protein, carbohydrates, and fats) using established conversion factors:
Protein: 4 calories per gram
Carbohydrates: 4 calories per gram
Fat: 9 calories per gram - Vitamin and Mineral Content: The amounts of vitamins and minerals are determined directly from the component analysis results and expressed as a percentage of the Daily Value (%DV) per serving.
- Example: Consider a breakfast cereal. Component examination might reveal that a 1-cup serving contains 30 grams of carbohydrates, 4 grams of protein, and 2 grams of fat. Using the above conversion factors, the total calories from macronutrients can be calculated as:
(30 grams carbohydrates x 4 calories/gram) + (4 grams protein x 4 calories/gram) + (2 grams fat x 9 calories/gram) = 188 calories
This value, along with the amounts of vitamins and minerals determined through analysis, is then presented in the nutritional facts panel.
Common Components and Their Evaluations
Hello! Let’s delve into the core building blocks of food and how we analyze them. Understanding these components – proteins, carbohydrates, fats, and water – is crucial for ensuring food safety, quality, and nutritional value. We’ll explore the specific methods used to assess each of these, and how these evaluations directly impact what we eat.
Proteins: Evaluation Methods
Proteins are essential for growth, repair, and various bodily functions. Evaluating protein content and quality is a critical aspect of food analysis. Several methods are employed for this purpose:* Kjeldahl Method: This is a widely used, official method for determining the total nitrogen content in a sample. The nitrogen content is then converted to protein content using a conversion factor (e.g., 6.25 for most foods, based on the assumption that protein contains approximately 16% nitrogen).
The process involves digestion, distillation, and titration.
Dumas Method (Combustion Method)
This method also measures total nitrogen content but utilizes high-temperature combustion. The sample is burned, and the released gases (including nitrogen) are measured. This method is faster and requires less chemical manipulation than the Kjeldahl method.
Amino Acid Analysis
This method involves hydrolyzing the protein to its constituent amino acids and then separating and quantifying them using chromatography, such as high-performance liquid chromatography (HPLC). This provides detailed information about the amino acid profile, which is essential for assessing protein quality.
Spectrophotometric Methods
These methods, like the Bradford assay or the Lowry assay, rely on colorimetric reactions with specific reagents that bind to proteins, producing a colored complex. The intensity of the color is proportional to the protein concentration and is measured using a spectrophotometer. These methods are often used for rapid protein quantification in research settings.
Carbohydrates: Evaluation Methods
Carbohydrates provide the body with energy. Evaluating carbohydrate content and types is important for understanding the nutritional profile of a food.* Total Carbohydrate Determination: This can be calculated by difference. The percentages of moisture, protein, fat, and ash are determined, and the total is subtracted from 100%.
Specific Sugar Analysis
Individual sugars (glucose, fructose, sucrose, lactose, etc.) are often quantified using chromatographic techniques, such as HPLC, gas chromatography (GC), or thin-layer chromatography (TLC). These methods allow for the separation and identification of different sugars.
Fiber Determination
Dietary fiber is analyzed using various methods, including enzymatic-gravimetric methods, such as the AOAC methods. These methods involve enzymatic digestion of the sample to remove starch and other digestible components, followed by filtration and weighing of the remaining fiber.
Starch Analysis
Starch can be quantified using enzymatic methods that convert starch to glucose, which is then measured using enzymatic or chromatographic techniques.
Fats: Evaluation Methods
Fats are essential for energy storage and absorption of fat-soluble vitamins. Evaluating fat content and composition is vital for food quality and nutritional assessment.* Soxhlet Extraction: This is a classic method for determining total fat content. The food sample is extracted with an organic solvent (e.g., petroleum ether) in a Soxhlet apparatus. The solvent dissolves the fat, which is then evaporated, and the remaining fat is weighed.
Acid Hydrolysis
This method is used to release bound fats, such as those found in dairy products. The sample is treated with acid to hydrolyze the fat-protein complex, allowing for extraction.
Chromatographic Techniques
Gas chromatography (GC) and high-performance liquid chromatography (HPLC) are used to analyze the fatty acid composition of fats. These techniques separate the fatty acids based on their physical and chemical properties, allowing for the identification and quantification of individual fatty acids.
Refractive Index
The refractive index of a fat can be measured using a refractometer. This value can be used to assess the purity and degree of unsaturation of the fat.
Water: Evaluation Methods
Water is a crucial component of food, influencing texture, stability, and shelf life. Evaluating water content is essential for food safety and quality control.* Drying Methods: These methods involve removing water from the sample by heating it in an oven until a constant weight is achieved. The weight loss represents the moisture content. Different temperatures and drying times are used depending on the food type.
Karl Fischer Titration
This is a precise method for determining water content. It involves reacting the water in the sample with a Karl Fischer reagent, and the amount of reagent consumed is directly proportional to the water content.
Distillation Methods
Notice pro pac cat food for recommendations and other broad suggestions.
The water is distilled from the sample, often with a solvent like toluene, and collected. The volume of water collected is then measured.
Water Activity (aw) Measurement
This is a measure of the “free” water available for microbial growth and chemical reactions. It is measured using a water activity meter.
Impact on Food Quality and Shelf Life
The evaluation of these components has a significant impact on food quality and shelf life. For instance:* Protein: Protein content and quality directly affect the nutritional value of food. High-quality protein is crucial for muscle building and repair. Protein degradation can lead to off-flavors and changes in texture, reducing shelf life.
Carbohydrates
The type and amount of carbohydrates impact the sweetness, texture, and energy content of food. Starch content influences the texture of baked goods, while the presence of sugars affects the browning reaction during cooking.
Fats
Fat content and composition influence the flavor, texture, and stability of food. The presence of unsaturated fats can lead to rancidity, which degrades the food and reduces its shelf life.
Water
Water content and water activity directly affect the texture, microbial stability, and shelf life of food. High water activity promotes microbial growth and spoilage.
Role of Water Activity in Food Preservation
Water activity (aw) plays a crucial role in food preservation. Controlling water activity is a primary strategy for preventing microbial spoilage and extending shelf life. Here’s a breakdown:* Microbial Growth: Microorganisms require a certain level of water activity to grow and multiply. Bacteria generally require a high aw (above 0.90), while yeasts and molds can tolerate lower aw values.
Chemical Reactions
Water activity also influences the rate of chemical reactions that can degrade food quality, such as lipid oxidation and enzymatic browning.
Preservation Techniques
Drying
Reducing water content (and thus, aw) through techniques like sun-drying, freeze-drying, or oven-drying inhibits microbial growth.
Adding Solutes
Adding solutes like sugar or salt lowers the aw by binding water molecules, making them unavailable for microbial use.
Freezing
Freezing converts water to ice, making it unavailable for microbial growth and slowing down chemical reactions.
Packaging
Packaging materials that provide a barrier to moisture can help maintain a low aw and extend shelf life.
For example, jerky has a low water activity, which inhibits the growth of microorganisms and extends its shelf life.
Emerging Trends in Component Evaluations
Hello again, food enthusiasts! We’ve journeyed through the fascinating world of food component analysis, from the fundamentals to the regulatory landscape. Now, let’s peek into the future! The field is dynamic, constantly evolving with cutting-edge technologies and methodologies. This exploration will reveal how innovation is reshaping how we understand, assess, and ensure the safety and quality of our food supply.
New Technologies and Methods in Component Evaluations
Advancements in technology are rapidly transforming component evaluations. Several novel approaches are emerging, enhancing the speed, precision, and scope of analysis. These include techniques that offer non-destructive testing, enabling the assessment of food components without compromising the sample. The integration of artificial intelligence and machine learning further accelerates data analysis and interpretation.
- Spectroscopic Techniques: These methods analyze how food interacts with light to identify and quantify components. Techniques such as Raman spectroscopy and hyperspectral imaging are gaining traction.
- Omics Technologies: Genomics, proteomics, and metabolomics provide comprehensive insights into the molecular makeup of food. These approaches enable the identification of a wide range of compounds, including allergens, contaminants, and nutritional components.
- Nanotechnology: Nanomaterials are utilized for advanced sensing and detection of food components. Nanoparticles can enhance the sensitivity and selectivity of analytical methods.
- Microfluidic Devices: These miniaturized devices offer rapid and efficient analysis. They can be used for on-site testing and point-of-care diagnostics, particularly in food safety.
Efficiency and Accuracy Improvements through New Techniques
The application of these new techniques has significantly improved efficiency and accuracy in component evaluations. The ability to perform analyses faster, with greater precision, and often with minimal sample preparation, is a game-changer. This leads to quicker results, more reliable data, and ultimately, better decision-making in food production and quality control.
- Rapid Analysis: Spectroscopic methods can analyze samples in seconds, drastically reducing the time needed for component identification.
- High Sensitivity: Nanotechnology-based sensors can detect trace amounts of contaminants, such as pesticides or toxins, with unprecedented sensitivity.
- Non-Destructive Testing: Techniques like hyperspectral imaging allow for the assessment of internal characteristics of food products without damaging them, providing valuable insights into quality and ripeness. For instance, this could mean assessing the sugar content of a mango without cutting it open.
- Improved Data Analysis: The use of AI and machine learning facilitates the automated analysis of large datasets, improving the accuracy and speed of identifying patterns and trends in food composition.
Impact of Trends on Food Safety and Quality Control
The rise of these innovative techniques is having a profound impact on food safety and quality control. These advancements enable more proactive and effective monitoring of food production, reducing the risk of contamination and ensuring that consumers receive safe and high-quality products.
- Enhanced Contaminant Detection: Advanced methods can identify and quantify a wider range of contaminants, including allergens, pathogens, and toxins, at lower levels.
- Improved Quality Assessment: Non-destructive techniques allow for a more comprehensive evaluation of product quality, including factors such as freshness, ripeness, and nutrient content.
- Traceability and Authenticity: New methods can be used to verify the origin and authenticity of food products, protecting consumers from fraud and ensuring that they receive what they pay for.
- Faster Response Times: Rapid analysis techniques enable quicker detection of problems in the food supply chain, allowing for faster responses to food safety incidents.
A groundbreaking method, employing a sophisticated combination of mass spectrometry and advanced chromatographic separation techniques, is emerging. This approach, coupled with sophisticated data analysis algorithms, allows for the simultaneous detection and quantification of hundreds of different compounds within a single food sample. This technology, by providing detailed compositional profiles, offers a holistic view of the food matrix. This level of detail helps in identifying potential adulteration, detecting contaminants, and assessing nutritional content with unprecedented accuracy. The method’s high sensitivity and throughput facilitate rapid and comprehensive food analysis, thus contributing significantly to food safety and quality control.
Practical Applications and Case Studies
Component evaluations are not just theoretical exercises; they are essential tools with tangible impacts on the food industry. Their application spans from preventing food safety crises to driving innovation in product development and safeguarding the authenticity of our food supply. This section delves into real-world examples to illustrate the practical significance of these evaluations.
Case Studies in Food Safety Incidents
Food safety incidents highlight the critical importance of component evaluations. When these evaluations fail, the consequences can be severe, ranging from consumer illness to widespread recalls and damage to brand reputation.The following examples showcase how component evaluations play a crucial role in identifying and mitigating food safety risks:
- The Peanut Corporation of America (PCA) Salmonella Outbreak (2008-2009): This devastating outbreak, which sickened over 700 people and resulted in nine deaths, stemmed from Salmonella contamination in peanut products. Failure to adequately test raw ingredients and finished products for Salmonella, alongside poor manufacturing practices, allowed contaminated products to reach consumers. Component evaluations, specifically microbial testing, could have identified the contamination before it spread.
- Melamine Contamination in Infant Formula (China, 2008): Melamine, a nitrogen-rich industrial chemical, was intentionally added to infant formula to artificially inflate protein content during testing. This fraudulent practice led to kidney failure in thousands of infants. Comprehensive chemical component evaluations, including chromatography and mass spectrometry, could have detected the presence of melamine and prevented this tragedy. The case underscored the importance of verifying ingredient authenticity and the devastating impact of fraudulent practices.
- E. coli Outbreaks in Leafy Greens: Recurrent outbreaks of E. coli linked to leafy greens, such as spinach and lettuce, often result from contamination during the growing, harvesting, or processing stages. Component evaluations, including microbiological testing and source tracking, are vital to identify the source of contamination and prevent future outbreaks. Analyzing the microbial profile of the produce and the surrounding environment is crucial.
Component Evaluations in Product Development
Component evaluations are instrumental in the development of new food products, enhancing their nutritional profile, flavor, texture, and shelf life. They provide crucial data for formulating recipes, selecting ingredients, and optimizing processing techniques.Here are some applications of component evaluations in product development:
- Nutritional Enhancement: Food manufacturers utilize component evaluations to determine the nutritional content of ingredients and finished products. This information is used to fortify foods with vitamins, minerals, or other beneficial compounds, as seen in the development of fortified cereals and functional foods. For instance, assessing the bioavailability of added iron in a cereal product is a component evaluation.
- Flavor and Texture Optimization: Component evaluations, including sensory analysis and instrumental measurements, help refine the flavor and texture of food products. For example, analyzing the volatile compounds in coffee beans to optimize roasting profiles or measuring the viscosity of a sauce to achieve the desired mouthfeel are critical.
- Shelf-Life Extension: Understanding the chemical and physical properties of food components is essential for extending shelf life. Evaluating the antioxidant content of a product to prevent rancidity or assessing the water activity to control microbial growth are examples of this application.
- Ingredient Substitution: Component evaluations allow for informed ingredient substitution. When a key ingredient becomes unavailable or too expensive, manufacturers can use component evaluations to compare the properties of alternative ingredients, ensuring the final product maintains its desired characteristics.
Ensuring Food Authenticity through Component Evaluations
Food fraud, including adulteration and mislabeling, is a persistent problem in the food industry. Component evaluations are indispensable tools for verifying the authenticity of food products and protecting consumers from deceptive practices.The following are ways component evaluations contribute to food authenticity:
- Origin Determination: Isotope ratio mass spectrometry (IRMS) can be used to determine the geographic origin of foods like honey, olive oil, and wine. This helps to identify products that are falsely labeled with a specific origin.
- Species Identification: DNA barcoding and protein analysis are employed to identify the species of origin in meat, fish, and other products. This helps to detect the substitution of less expensive or undeclared species.
- Ingredient Verification: Component evaluations, such as chromatography and spectroscopy, can detect the presence of undeclared ingredients or contaminants. For instance, detecting the addition of undeclared vegetable oils in olive oil or the presence of artificial colors in honey.
- Detection of Adulteration: Chemical analysis can identify adulterants added to increase profit margins. Examples include the addition of water to milk, sugar to honey, or cheaper oils to more expensive oils.
Visual Representation: Case Study – Component Examination Failure and Impact
A visual representation, such as a flowchart or diagram, can effectively illustrate the impact of a component examination failure. This example focuses on a hypothetical scenario of a contaminated batch of baby food.The visual representation would include these stages:
1. Production Process
Starts with the sourcing of raw ingredients (e.g., fruits, vegetables, meat).
2. Component Examination
The raw ingredients are subjected to component examinations (e.g., microbial testing, pesticide residue analysis).
3. Examination Failure
The visual representation shows a breakdown at the component examination stage. For example, the microbial testing fails to detect the presence ofSalmonella* in a batch of fruit puree.
4. Production Continues
Despite the examination failure, the contaminated fruit puree is used in the production of baby food.
5. Packaging and Distribution
The contaminated baby food is packaged and distributed to retail stores.
6. Consumer Consumption
Consumers purchase and feed the contaminated baby food to infants.
7. Adverse Health Effects
Infants become ill with symptoms of salmonellosis (e.g., fever, diarrhea, vomiting).
8. Recall and Investigation
Cases of illness are reported, leading to a product recall and investigation.
9. Consequences
The representation illustrates the consequences:
- Infant illness and potential hospitalization.
- Damage to the manufacturer’s reputation.
- Financial losses due to recall and legal action.
The visual would also highlight the importance of robust component examinations and the devastating consequences of failure.
Conclusion
In conclusion, food ingredient analysis is a dynamic field, constantly evolving with technological advancements and emerging challenges. It serves as a cornerstone for food safety, quality control, and consumer trust. From the simple starch test to sophisticated chromatographic techniques, the methods are diverse and powerful. By embracing these practices and staying informed about the latest trends, we can empower consumers, support responsible food production, and build a healthier, more transparent food system.
The future of our food depends on a continuous commitment to rigorous ingredient analysis.