Blank food web, a whisper in the wind, a canvas waiting for the strokes of life. Imagine a world unveiled, a stage set for the drama of existence, where every creature, from the smallest microbe to the tallest tree, plays a vital role. This isn’t just a scientific diagram; it’s a portal, a key to understanding the intricate web that binds us all.
Prepare to delve into the unseen, where energy flows like a hidden river, shaping the very fabric of our planet.
A blank food web, at its core, is a foundational tool in ecological studies. It serves as a starting point, a blank slate upon which we can sketch the relationships between organisms. Producers, the sun’s alchemists, transform light into sustenance. Consumers, the diners, feast upon this bounty, and decomposers, the silent recyclers, return life to the earth. By starting with this framework, we can then populate it with real-world examples, building complex models to understand how ecosystems function.
Introduction to Blank Food Webs
The dance of life, a symphony of interconnectedness, is often best understood through the lens of a food web. But before we can appreciate the complex choreography of ecosystems, we need a starting point, a blank canvas upon which to paint the intricate relationships of life. This is where the blank food web enters, a foundational tool for ecological exploration.A blank food web, in its essence, is a simplified, often schematic, representation of the feeding relationships within an ecosystem.
It provides a framework for understanding how energy and nutrients flow through an environment. It’s a conceptual map, devoid of specific species or detailed interactions initially, allowing for a focused exploration of the fundamental principles governing ecological dynamics.
Defining Food Webs and Their Components
A food web illustrates the interconnected feeding relationships within an ecological community. It is not a simple, linear chain, but a complex network where organisms are linked by who eats whom.The core components of a food web are:
- Producers: These are the autotrophs, the “self-feeders.” They are the foundation of the food web, primarily plants, algae, and some bacteria, that convert sunlight into energy through photosynthesis. They harness the sun’s power to create organic molecules, forming the base of the food chain.
- Consumers: These are the heterotrophs, organisms that obtain their energy by consuming other organisms. They can be categorized based on their diet:
- Primary Consumers (Herbivores): They eat producers. Examples include deer grazing on grass, caterpillars munching on leaves, and krill feeding on phytoplankton.
- Secondary Consumers (Carnivores/Omnivores): They eat primary consumers. Examples include wolves preying on deer, frogs eating insects, and humans consuming both plants and animals.
- Tertiary Consumers (Apex Predators): They eat secondary consumers. Examples include lions hunting wolves, sharks consuming smaller fish, and eagles preying on rabbits.
- Decomposers: These are the recyclers, the essential organisms that break down dead organic matter (detritus) and return nutrients to the environment. Examples include bacteria, fungi, and certain invertebrates like earthworms. They complete the cycle of life by converting dead organisms and waste into simpler substances that producers can utilize.
Significance of Blank Food Webs in Ecological Modeling
The power of a blank food web lies in its flexibility and adaptability. It serves as an essential starting point for creating more complex ecological models, allowing researchers to explore various scenarios and hypotheses.Blank food webs offer several advantages:
- Simplification: They allow ecologists to focus on fundamental principles without being overwhelmed by the complexity of a real-world ecosystem. By stripping away specific species, the basic flow of energy and nutrients becomes more apparent.
- Conceptual Framework: They provide a framework for understanding how energy and nutrients move through an ecosystem. This framework is useful for understanding the impacts of removing or adding a species to the web.
- Scenario Testing: Blank food webs can be used to simulate the effects of environmental changes, such as habitat loss, pollution, or climate change. By manipulating the components, researchers can predict how these changes might impact the ecosystem.
- Building Complexity: The blank food web can be progressively filled with species-specific data, creating more detailed and realistic models. This allows researchers to explore the intricate interactions within a specific ecosystem.
For example, consider a hypothetical marine ecosystem. A blank food web might initially depict producers (phytoplankton), primary consumers (zooplankton), secondary consumers (small fish), and tertiary consumers (larger fish). The blank web allows for the easy insertion of specific species, such as adding “tuna” as a tertiary consumer that preys on “mackerel,” a secondary consumer. Further complexity can be added by including decomposers and detailing the energy transfer rates between trophic levels.
This iterative process enables researchers to build increasingly sophisticated models that reflect the intricate realities of an ecosystem.
Identifying Organisms and Trophic Levels
The creation of a blank food web necessitates the careful selection and categorization of organisms. This process involves understanding the roles organisms play within an ecosystem and how energy flows between them. This section details the identification of suitable organisms and their classification into distinct trophic levels, forming the foundation for a functional food web.
Identifying Organisms for a Blank Food Web
The initial step involves identifying a diverse range of organisms from various ecosystems to populate the blank food web. This selection should encompass a spectrum of life forms, ensuring representation across different trophic levels. Consider the following examples, drawn from diverse environments:
- Aquatic Ecosystems: Selecting organisms from aquatic environments provides a rich array of possibilities. For example, phytoplankton, microscopic algae that form the base of many aquatic food webs, can be included as primary producers. Zooplankton, tiny animals that consume phytoplankton, serve as primary consumers. Small fish, such as minnows, that eat zooplankton are secondary consumers. Larger predatory fish, such as bass, that feed on the smaller fish represent tertiary consumers.
- Terrestrial Ecosystems: Terrestrial ecosystems offer an equally diverse selection of organisms. Plants, like grasses and trees, are primary producers. Herbivores, such as deer and rabbits, act as primary consumers, feeding on the plants. Carnivores, such as foxes and wolves, that prey on the herbivores function as secondary and tertiary consumers. Decomposers, such as fungi and bacteria, break down dead organic matter, recycling nutrients back into the ecosystem.
- Desert Ecosystems: Even harsh environments like deserts offer a range of organisms. Cacti and other desert plants serve as primary producers. Herbivores like desert rodents and insects consume the plants. Snakes and other predators prey on the herbivores, acting as secondary consumers. Scavengers like vultures contribute to nutrient cycling by consuming carrion.
Classifying Organisms into Trophic Levels
Once organisms are identified, the next step is classifying them into their respective trophic levels. This categorization reflects the flow of energy through the food web. The following levels are typically recognized:
- Primary Producers: These are autotrophic organisms, such as plants, algae, and some bacteria, that generate their own food through photosynthesis or chemosynthesis. They form the base of the food web.
- Primary Consumers (Herbivores): These organisms consume primary producers. They obtain energy by eating plants or algae. Examples include grasshoppers, deer, and zooplankton.
- Secondary Consumers (Carnivores/Omnivores): These organisms consume primary consumers. They obtain energy by eating herbivores. Examples include foxes, snakes, and some fish.
- Tertiary Consumers (Apex Predators): These are top-level predators that consume secondary consumers. They are typically at the top of the food web and are not usually preyed upon. Examples include wolves, eagles, and sharks.
- Decomposers: These organisms, such as fungi and bacteria, break down dead organic matter (detritus) and recycle nutrients back into the ecosystem. They play a crucial role in nutrient cycling.
The classification of organisms into trophic levels is a fundamental principle in ecology, reflecting the flow of energy and nutrients through an ecosystem. Understanding these levels allows for a comprehensive understanding of the interactions between organisms and the overall health of the environment.
Example Organisms Categorized by Trophic Level
To illustrate the classification process, consider a hypothetical blank food web. Here is a list of example organisms, categorized by trophic level:
- Primary Producers:
- Grasses
- Trees (e.g., oak, pine)
- Phytoplankton (e.g., diatoms, green algae)
- Primary Consumers:
- Deer
- Rabbits
- Zooplankton (e.g., Daphnia)
- Grasshoppers
- Secondary Consumers:
- Foxes
- Snakes
- Small Fish (e.g., minnows)
- Tertiary Consumers:
- Wolves
- Eagles
- Larger Fish (e.g., bass)
- Decomposers:
- Fungi (e.g., mushrooms)
- Bacteria (e.g.,
-Escherichia coli*)
Constructing a Basic Blank Food Web
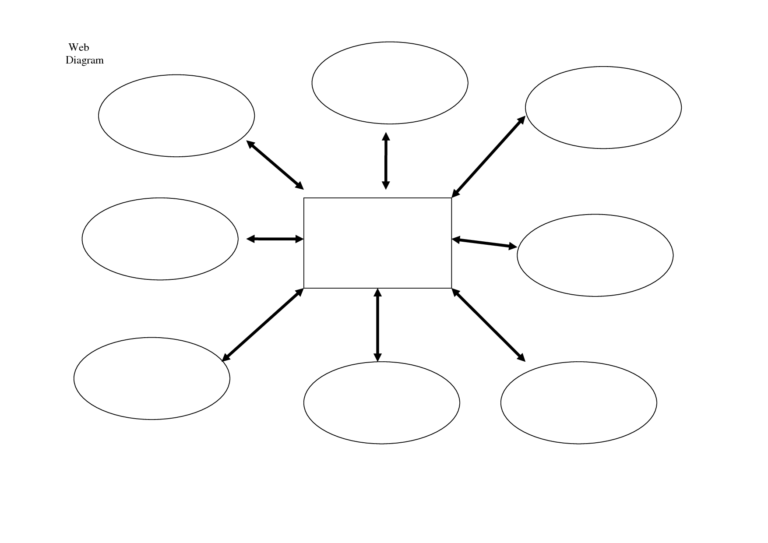
To truly grasp the intricate dance of life within an ecosystem, we must learn to weave the threads of connection that bind organisms together. Constructing a blank food web allows us to visualize these relationships, revealing the flow of energy and the roles each creature plays. This exercise, though seemingly simple, lays the groundwork for understanding complex ecological dynamics.
Step-by-Step Procedure for Constructing a Blank Food Web
The creation of a blank food web is a methodical process, built upon careful observation and understanding of organismal roles. It begins with identification and culminates in a visual representation of energy transfer.
- Identify the Organisms: The initial step involves identifying the organisms that inhabit the ecosystem you’re studying. This could range from a microscopic world to a vast forest.
- List all the species you observe or have information about. For example, in a pond ecosystem, this might include algae, water fleas, small fish, frogs, and herons.
- Determine Trophic Levels: Categorize each organism based on its feeding habits, placing them into appropriate trophic levels.
- Producers (autotrophs) form the base, typically plants or algae.
- Primary consumers (herbivores) eat producers.
- Secondary consumers (carnivores or omnivores) eat primary consumers.
- Tertiary consumers (top predators) eat secondary consumers, and so on.
- Establish Feeding Relationships: Research and document which organisms consume which. This is the heart of constructing the web.
- Consider what each organism eats. Algae are consumed by water fleas; small fish eat water fleas; frogs eat small fish; and herons eat frogs.
- Draw Arrows to Represent Energy Flow: Using arrows, visually depict the direction of energy flow. The arrow points from the organism being eaten to the organism doing the eating.
- An arrow from algae to a water flea indicates that the water flea consumes algae.
- Refine and Expand: As you gather more information, refine your food web. Add more organisms, and detail the complexity of the relationships.
- Consider the omnivores and the various food sources of each organism to enrich the web.
Demonstrating the Process of Drawing Arrows to Represent Energy Flow
Arrows are the language of a food web, the visual indicators that trace the path of energy transfer. Understanding how to use them correctly is critical for interpreting the web’s meaning.The direction of the arrow is paramount. It always points from the organism being consumed to the consumer. This illustrates the flow of energy. For example, if a caterpillar eats a leaf, the arrow goes from the leaf to the caterpillar.
This simple convention immediately reveals who eats whom. The density and direction of these arrows reveal the web’s complexity. A web with many arrows demonstrates a complex system, while a web with fewer arrows is a simpler one.Consider a hypothetical meadow. The grass is the producer. A grasshopper eats the grass (arrow from grass to grasshopper).
A bird eats the grasshopper (arrow from grasshopper to bird). A fox eats the bird (arrow from bird to fox). The arrows clearly show the energy’s journey.
Example of a Simple, Basic Blank Food Web
A simplified food web, though limited in scope, reveals the core principles of energy flow. The example presented illustrates these principles in a concise manner.Let’s consider a simple pond ecosystem. We will include four organisms and three trophic levels.
Organism | Trophic Level | Feeding Relationship |
---|---|---|
Algae | Producer | (Makes its own food through photosynthesis) |
Water Fleas | Primary Consumer | Eats Algae |
Small Fish | Secondary Consumer | Eats Water Fleas |
Heron | Tertiary Consumer | Eats Small Fish |
The food web representation would look like this:
Algae -> Water Fleas -> Small Fish -> Heron
This basic example, despite its simplicity, effectively demonstrates the flow of energy from the producer (algae) through the consumers, ultimately to the top predator (heron).
Ecosystem Types and Blank Food Web Variations
Blank food webs, in their adaptability, serve as valuable tools for understanding the intricate relationships within diverse ecosystems. They allow for a simplified representation of energy flow, trophic interactions, and the potential impacts of disturbances within different environmental contexts. The following sections explore how blank food webs can be tailored to reflect the unique characteristics of various ecosystem types.
Adapting Blank Food Webs for Terrestrial and Aquatic Ecosystems
Blank food webs can be modified to represent a wide array of ecosystems, from terrestrial forests to aquatic marine environments. The key lies in selecting the appropriate organisms and trophic levels relevant to the specific ecosystem being modeled.To illustrate, consider the following points:
- Terrestrial Ecosystems: A blank food web for a forest ecosystem might include primary producers like trees, shrubs, and grasses. Primary consumers would encompass herbivores such as deer, rabbits, and insects. Secondary consumers could be predators like foxes, wolves, and birds of prey. Decomposers, including fungi and bacteria, would break down organic matter.
- Aquatic Ecosystems: In contrast, a marine ecosystem’s blank food web would feature primary producers like phytoplankton and macroalgae. Primary consumers would include zooplankton and small filter-feeding organisms. Secondary consumers would consist of fish, marine mammals, and larger invertebrates. Decomposers, such as bacteria, would break down organic matter in the water and on the seafloor.
Comparing Trophic Structures: Forest vs. Marine Ecosystems
The typical organisms and trophic structures of a forest ecosystem and a marine ecosystem differ significantly due to the fundamental differences in their environmental conditions and resource availability.Here’s a comparative analysis:
Feature | Forest Ecosystem | Marine Ecosystem |
---|---|---|
Primary Producers | Trees, shrubs, grasses | Phytoplankton, macroalgae (seaweed) |
Primary Consumers | Herbivores (deer, rabbits, insects) | Zooplankton, filter feeders (e.g., krill, some shellfish) |
Secondary Consumers | Carnivores (foxes, wolves, birds of prey) | Fish, marine mammals, larger invertebrates (e.g., sharks, seals, squid) |
Decomposers | Fungi, bacteria | Bacteria, other microorganisms |
Energy Source | Sunlight, soil nutrients | Sunlight, dissolved nutrients in the water |
The primary producers in a forest are rooted plants that obtain energy from sunlight and nutrients from the soil. In contrast, marine ecosystems’ primary producers are primarily phytoplankton, microscopic organisms that drift in the water and use sunlight for photosynthesis, and macroalgae.
Challenges of Blank Food Webs in Complex Ecosystems
Creating blank food webs for highly complex ecosystems presents significant challenges. Ecosystems like coral reefs, with their immense biodiversity and intricate interactions, require careful consideration and simplification to remain manageable and informative.The difficulties include:
- High Biodiversity: Coral reefs support an extraordinary number of species, making it challenging to represent all the organisms and their interactions within a manageable food web.
- Complex Interactions: The relationships between organisms in coral reefs are highly complex, including symbiotic relationships, intricate predator-prey dynamics, and competitive interactions. Accurately depicting these requires careful selection and simplification.
- Data Availability: Obtaining comprehensive data on the feeding habits and trophic levels of all reef organisms can be difficult, limiting the accuracy of the food web.
- Spatial Heterogeneity: Coral reefs exhibit significant spatial variation, with different habitats and species distributions. Creating a single food web that accurately represents the entire reef ecosystem is challenging.
Despite these challenges, blank food webs remain valuable tools for understanding coral reef ecosystems. By focusing on key species and trophic levels, these models can help researchers and conservationists identify vulnerabilities and assess the potential impacts of environmental stressors such as climate change, pollution, and overfishing. For instance, a blank food web could highlight the importance of specific keystone species, such as sharks or certain herbivorous fish, and illustrate how their removal could trigger cascading effects throughout the reef ecosystem.
Adding Complexity: Species Interactions
The elegance of a blank food web lies in its simplicity, but ecosystems are rarely simple. To truly represent the intricate dance of life, we must introduce the complexities of species interactions. This involves depicting not just “who eats whom,” but also how species compete, cooperate, and form relationships that shape the ecosystem. Adding these nuances transforms a basic food web into a powerful tool for understanding ecological dynamics.
Representing Complex Interactions, Blank food web
Incorporating complex species interactions necessitates a more sophisticated approach to visual representation. While the simple arrow indicating energy flow is fundamental, it’s insufficient to capture the full picture. Different graphical conventions and annotations are needed to depict various interactions.
Omnivory, Competition, and Symbiosis
Omnivory, the consumption of multiple trophic levels, can be illustrated by arrows originating from the omnivore and pointing towards all of its food sources. Competition, a struggle for resources, can be shown through dashed lines or intersecting arrows between competing species. Symbiosis, a close and often long-term interaction between different biological species, demands specific visual representations depending on the type of relationship.
Symbiotic Relationships in Food Web Diagrams
Symbiotic relationships are crucial in understanding ecosystem function. These relationships, which involve two or more species living in close proximity, can be broadly categorized.
- Mutualism: Both species benefit from the interaction. This can be shown with a double-headed arrow between the species, signifying a reciprocal exchange of resources or services. For instance, in a coral reef ecosystem, coral polyps (animals) and zooxanthellae (algae) have a mutualistic relationship. The algae provide the coral with food through photosynthesis, while the coral provides the algae with a protected environment and access to sunlight.
This interaction is vital for the reef’s health.
- Commensalism: One species benefits, and the other is neither harmed nor helped. This is typically represented by an arrow pointing from the commensal species to the benefiting species, with a notation (e.g., “+0”) to indicate the neutral impact on the other. An example is the relationship between barnacles and whales. Barnacles attach themselves to whales, gaining access to a mobile habitat and food sources, while the whales are generally unaffected.
- Parasitism: One species (the parasite) benefits at the expense of the other (the host). This is depicted by an arrow pointing from the host to the parasite, often with a notation (e.g., “+-“) to indicate the detrimental effect on the host. Ticks feeding on a mammal would be an example of parasitism. The tick obtains nourishment, while the mammal suffers blood loss and potential disease transmission.
Graphical Representations of Common Species Interactions
To visually clarify the nature of species interactions, consistent graphical conventions are essential.
- Predation: Solid arrow from prey to predator (e.g., grasshopper → bird).
- Competition: Dashed lines or intersecting arrows between competing species (e.g., two species of deer competing for the same food source).
- Mutualism: Double-headed arrow between interacting species (e.g., pollinator ↔ plant).
- Commensalism: Arrow from commensal to the benefiting species, with a notation (e.g., “+0”) (e.g., remora → shark).
- Parasitism: Arrow from host to parasite, with a notation (e.g., “+-“) (e.g., tick → dog).
- Omnivory: Arrows from the omnivore to multiple food sources across different trophic levels (e.g., a bear consuming berries, fish, and insects).
Representing Energy Flow and Trophic Efficiency
In the intricate dance of life within a blank food web, energy flows, a vital current driving all interactions. Understanding this flow, and its inherent inefficiencies, is crucial to comprehending the dynamics of any ecosystem. This section explores how to visualize this energy transfer and the principles that govern it.
Illustrating Energy Flow
Energy, the lifeblood of any ecosystem, moves unidirectionally through a food web, starting with primary producers and cascading upwards. Representing this flow visually helps to grasp the relationships between organisms and the dependencies within the web.To effectively illustrate energy flow, several methods can be employed:
- Arrows: Arrows are the most fundamental visual tool. They are drawn from the organism being consumed (the prey) to the organism consuming it (the predator). The direction of the arrow unequivocally indicates the direction of energy transfer. A plant is eaten by a caterpillar; the arrow points from the plant to the caterpillar, showing energy is moving from the plant to the caterpillar.
- Color Coding: Color can represent different trophic levels. For example, primary producers (plants) could be green, primary consumers (herbivores) yellow, secondary consumers (carnivores) orange, and tertiary consumers (top predators) red. This visual distinction helps to quickly identify the position of each organism in the energy flow.
- Size Representation: The size of the organism or its representation can correspond to the amount of energy it contains or the amount of biomass it represents. Larger organisms, or representations of them, would indicate a greater energy storage capacity.
- Energy Pyramids: Energy pyramids are a powerful visual representation of energy flow and trophic levels. The pyramid shape illustrates that energy decreases at each successive trophic level. The base of the pyramid, representing primary producers, is the widest, and each subsequent level narrows, reflecting the loss of energy.
Visualizing Biomass and Energy at Each Trophic Level
Quantifying the amount of biomass or energy at each trophic level provides a deeper understanding of ecosystem structure and function. Visual representations, such as pyramids, are commonly used.Here’s how to design a method to visually represent the relative biomass or energy:
- Energy Pyramids: As mentioned, energy pyramids are a standard tool. The width of each level of the pyramid is proportional to the amount of energy or biomass present at that trophic level. This visually represents the decreasing energy availability as you move up the food chain.
- Biomass Pyramids: Similar to energy pyramids, biomass pyramids depict the total mass of organisms at each trophic level. These pyramids can sometimes be inverted, particularly in aquatic ecosystems where primary producers (phytoplankton) may have a lower biomass than primary consumers (zooplankton) at any given time.
- Numbers Pyramids: These pyramids represent the number of individuals at each trophic level. While useful, they can be misleading, as a few large organisms may have a greater impact than a multitude of small ones.
- Data Tables: Alongside visual representations, data tables can provide specific values for energy, biomass, or numbers at each trophic level. This allows for a more precise understanding of the ecosystem’s energy dynamics.
Consider a simple grassland ecosystem:
Trophic Level | Organism | Energy (kJ/m²/year) |
---|---|---|
Producers | Grass | 10,000 |
Primary Consumers | Grasshoppers | 1,000 |
Secondary Consumers | Birds | 100 |
This data, when used to construct an energy pyramid, would clearly illustrate the decreasing energy availability at each trophic level.
The 10% Rule and Its Implications
Energy transfer between trophic levels is not perfectly efficient. A significant portion of the energy consumed at one level is lost through metabolic processes (like respiration), waste (like feces), and heat. The 10% rule encapsulates this principle.The 10% rule states that, on average, only about 10% of the energy from one trophic level is transferred to the next. The remaining 90% is lost as heat, used for metabolic processes, or remains in the form of undigested material.
The 10% rule: Only about 10% of the energy from one trophic level is transferred to the next.
Implications of the 10% rule are:
- Energy Limitations: The 10% rule explains why food chains are typically limited to four or five trophic levels. There is simply not enough energy available at the higher levels to support a large population.
- Biomass Reduction: The biomass of organisms decreases as you move up the trophic levels. This is a direct consequence of the energy loss.
- Ecosystem Stability: Understanding the 10% rule helps in predicting how changes at one trophic level will affect the entire ecosystem. For instance, the removal of a top predator can have cascading effects throughout the food web.
- Human Impact: The 10% rule also applies to human food chains. Eating lower on the food chain (e.g., plants) is more energy-efficient than eating higher on the food chain (e.g., meat). This is why vegetarian diets often have a smaller ecological footprint.
Illustrating a Blank Food Web with HTML Tables
The power of a food web lies not only in its conceptual representation but also in its practical application. Visualizing these complex relationships can be significantly enhanced through the use of HTML tables, providing a structured and accessible way to display information about organisms, their trophic levels, diets, and predator-prey interactions. This allows for a clearer understanding of the interconnectedness within an ecosystem.
Creating an HTML Table for a Blank Food Web
An HTML table provides a structured format for presenting the essential components of a blank food web. This approach offers a clear and organized method for displaying complex ecological relationships.To effectively illustrate a blank food web, an HTML table can be structured with the following columns:
Organism | Trophic Level | Diet | Primary Predators |
---|---|---|---|
A. Phytoplankton | Primary Producer | N/A (Photosynthesis) | B. Zooplankton |
B. Zooplankton | Primary Consumer | A. Phytoplankton | C. Small Fish |
C. Small Fish | Secondary Consumer | B. Zooplankton | D. Larger Fish |
D. Larger Fish | Tertiary Consumer | C. Small Fish | E. Apex Predator (e.g., Shark) |
E. Apex Predator (e.g., Shark) | Apex Predator | D. Larger Fish | N/A (Apex Predator) |
This table provides a clear overview of the relationships. The “Organism” column lists the species in the food web. The “Trophic Level” column indicates the feeding position of each organism. The “Diet” column specifies the food source, and the “Primary Predators” column identifies the main consumers of each organism.
Enhancing Readability with Visual Cues
Enhancing readability and understanding within the table is crucial. Color-coding and other visual cues can significantly improve the clarity of the information presented.Consider these methods to enhance readability:
- Color-coding Trophic Levels: Assigning different background colors to each trophic level can quickly highlight the feeding relationships. For example, primary producers (e.g., phytoplankton) could be light green, primary consumers (e.g., zooplankton) light blue, secondary consumers (e.g., small fish) light orange, and so on. This immediately visually differentiates the different roles within the food web.
- Highlighting Predator-Prey Relationships: Using a consistent text color or bolding the text in the “Diet” and “Primary Predators” columns can visually link predator and prey. For instance, the diet of the zooplankton, A. Phytoplankton, could be in bold and the primary predator, C. Small Fish, could be bolded as well. This instantly emphasizes the direct interactions between organisms.
- Font Styles and Sizes: Using different font styles or sizes for specific categories can also improve readability. For example, the “Organism” column could use a slightly larger font size or a different font style to distinguish the organism names from the rest of the information.
- Consistent Formatting: Maintain consistent formatting throughout the table. This includes using the same font, size, and alignment for all entries within a column, enhancing the overall visual coherence of the table.
Implementing these visual enhancements transforms the table from a simple data presentation tool into a dynamic and easily understandable representation of the food web’s complexity.
Illustrating a Blank Food Web with Blockquotes
The beauty of a food web lies in its intricate connections, a tapestry woven from the threads of life and sustenance. While visual representations are often preferred, we can capture the essence of these relationships through text, specifically using blockquotes to delineate trophic levels and represent feeding interactions. This method allows us to build a hierarchical structure, mimicking the flow of energy from one organism to another.
Representing Trophic Levels with Indentation
The effectiveness of a text-based food web hinges on a clear and consistent system of indentation. This visual cue mirrors the structure of the food web, with primary producers at the base and apex predators at the top.
Producers (e.g., plants, algae)
Primary Consumers (herbivores)
Secondary Consumers (carnivores, omnivores)
Tertiary Consumers (apex predators)
This structure demonstrates the fundamental principle of energy transfer. The deeper the indentation, the higher the trophic level, and the more dependent the organism is on the levels below it.
Example of a Text-Based Food Web Representation
Let’s construct a simplified food web, illustrating how blockquotes can bring it to life. Consider a hypothetical ecosystem with plants, rabbits, foxes, and hawks.
Plants (Producers)
Rabbits (Primary Consumers)
Foxes (Secondary Consumers)
Hawks (Tertiary Consumers/Apex Predators)
Examine how halal food in kissimmee fl can boost performance in your area.
Foxes (Secondary Consumers)
In this example:
- Plants, the producers, form the base of the food web.
- Rabbits, consuming the plants, are the primary consumers.
- Foxes, feeding on rabbits, are secondary consumers. Notice that foxes also have an alternate pathway, directly consuming the plants.
- Hawks, preying on foxes, occupy the highest trophic level in this simplified example.
This textual representation provides a clear overview of the feeding relationships. It highlights the direction of energy flow and the roles of each organism within the ecosystem. The blockquote structure emphasizes the hierarchical nature of the food web. This system can be expanded to include more species and complex interactions, always maintaining the clarity of indentation to visually separate the different trophic levels.
Dynamic Food Webs
Food webs are not static diagrams etched in stone; they are vibrant, breathing representations of interconnected life, constantly shifting in response to a multitude of factors. Understanding these dynamic qualities is crucial to appreciating the fragility and resilience of ecosystems. By incorporating disturbances into blank food webs, we gain the power to anticipate, analyze, and ultimately, better manage the complexities of our natural world.
Representing Environmental Disturbances
Environmental disturbances, from pollution to climate change, can have profound and cascading effects throughout a food web. Representing these impacts in a blank food web requires a flexible and adaptable approach. This involves considering the specific nature of the disturbance and its potential consequences for different species and trophic levels.
- Direct Impacts: Pollution, for instance, might directly poison a primary producer, such as algae, or a primary consumer, like a zooplankton species. In the blank food web, this can be visualized by either removing the affected species entirely or reducing the arrow size representing energy flow from that species.
- Indirect Impacts: Climate change, leading to altered temperatures and ocean acidification, can indirectly affect food webs. This could impact the calcification process of shellfish, a key food source for larger predators. This is represented by modifying the relationships between species, perhaps reducing the arrow size from shellfish to their predators.
- Cascading Effects: The removal or decline of a key species can initiate a cascade of consequences. Consider the introduction of a non-native invasive species that preys on a dominant primary consumer. This can lead to a decrease in the primary consumer population, which in turn can lead to an increase in the population of primary producers, altering the entire ecosystem structure.
This would be modeled by removing or diminishing the energy flow from the affected primary consumer to the primary producers and potentially increasing the flow from the primary producers, reflecting the altered trophic dynamics.
Modeling the Impact of Removing a Key Species
Removing a key species, a species that exerts a strong influence on the structure and function of an ecosystem, offers a powerful demonstration of the interconnectedness within a food web. A blank food web provides an ideal platform for simulating these effects.
- Apex Predators: Removing an apex predator, like a wolf or a shark, can lead to a trophic cascade. The prey of the apex predator may increase in population, leading to increased grazing pressure on primary producers. This can be represented by removing the arrows from the apex predator to its prey and then altering the arrows from the prey to their food sources to show increased consumption.
- Foundation Species: Removing a foundation species, like a kelp forest or a coral reef, can dramatically alter the entire ecosystem. This can be visualized by removing the species from the food web and considering the consequences for all the organisms that depend on it for food or habitat.
- Keystone Species: Removing a keystone species, a species whose impact on its ecosystem is disproportionately large relative to its abundance, can also cause dramatic shifts. An example is the sea otter, which controls sea urchin populations, thus protecting kelp forests. The removal of sea otters could lead to overgrazing of kelp forests by sea urchins, causing a decline in the kelp forest and impacting numerous other species.
Incorporating Dynamic Elements and Ecosystem Changes
Incorporating dynamic elements into a blank food web is essential to accurately reflect the constant state of flux in ecosystems. This requires a shift from a static representation to one that can be adapted and modified to simulate change over time.
- Time-Series Analysis: Representing changes over time can be done by creating a series of blank food webs that reflect the ecosystem at different points in time. This could be used to model the recovery of a forest after a wildfire, the spread of an invasive species, or the long-term effects of climate change.
- Population Dynamics: Incorporating population dynamics can add further realism. The size of the arrows representing energy flow can be made to vary over time to reflect changes in population size.
- Feedback Loops: Including feedback loops, where changes in one part of the food web influence other parts, allows for the simulation of complex interactions. For example, an increase in the population of a predator might lead to a decrease in its prey, which in turn might lead to a decline in the predator population, illustrating a negative feedback loop.
- Scenario Planning: Blank food webs can be used for scenario planning, allowing scientists and policymakers to explore the potential consequences of different management strategies. For example, a blank food web could be used to model the impact of different fishing quotas on a marine ecosystem.
Illustrative Examples of Blank Food Webs
The beauty of a blank food web lies in its adaptability, its ability to be molded and filled with the specifics of any environment. It allows us to strip away the complexity and focus on the fundamental relationships between organisms, from the smallest producer to the apex predator. Let’s explore two examples, one terrestrial and one aquatic, to illustrate how this framework can be applied to diverse ecosystems.
Terrestrial Blank Food Web: Grassland Ecosystem
Grasslands, vast stretches of open land dominated by grasses and herbaceous plants, support a rich tapestry of life. The blank food web of a grassland highlights the crucial links between its inhabitants, from the sun-soaked blades of grass to the soaring raptors that patrol the skies.
Here’s an overview of a typical grassland food web:
- Producers: The foundation of the grassland food web is, of course, the grasses themselves, such as bluestem, switchgrass, and buffalo grass. These autotrophs harness the sun’s energy through photosynthesis, converting it into the energy that fuels the entire ecosystem. Also, wildflowers like coneflowers and sunflowers contribute to the energy base.
- Primary Consumers (Herbivores): A diverse array of herbivores graze on the grasses and wildflowers. These include:
- Bison: Large herbivores that consume vast quantities of grass, playing a vital role in shaping the grassland landscape.
- Prairie Dogs: These burrowing rodents feed on grasses and other plants, creating underground tunnels that influence soil structure and provide habitat for other species.
- Crickets and Grasshoppers: These insects are a significant food source for many predators, while also consuming large amounts of plant matter.
- Secondary Consumers (Primary Carnivores): These carnivores feed on the herbivores:
- Coyotes: Apex predators in many grasslands, hunting prairie dogs, rabbits, and other herbivores.
- Snakes: Various snake species, such as the garter snake, prey on rodents and insects.
- Hawks and Owls: Birds of prey that hunt small mammals and other animals from above.
- Tertiary Consumers (Secondary Carnivores/Apex Predators): These are the top predators, often feeding on the secondary consumers.
- Coyotes (can also act as tertiary consumers): Depending on the food web dynamics, coyotes might prey on smaller carnivores.
- Eagles: Large birds of prey that hunt larger animals.
- Decomposers: Decomposers like bacteria and fungi break down dead organic matter (plants and animals), returning nutrients to the soil, which is essential for the producers.
The relationships within this grassland food web are dynamic. For instance, a decrease in the prairie dog population could lead to an increase in grass growth, impacting the food available for herbivores and shifting the balance of predator-prey relationships. The entire system is interconnected, and each species plays a crucial role.
Aquatic Blank Food Web: Freshwater Lake Ecosystem
Freshwater lakes, with their diverse aquatic life, present a different set of challenges and opportunities for the construction of a blank food web. The flow of energy here is primarily driven by the sun, which is then utilized by photosynthetic organisms.
Here’s a breakdown of a typical freshwater lake food web:
- Producers: The base of the food web is formed by:
- Phytoplankton: Microscopic, free-floating algae that utilize sunlight for photosynthesis.
- Aquatic Plants: Rooted plants like water lilies and pondweed that grow along the lake’s edges and in shallower areas.
- Primary Consumers (Herbivores): These feed directly on the producers:
- Zooplankton: Tiny, free-floating animals, such as copepods and daphnia, that graze on phytoplankton.
- Aquatic Insects: Larvae of insects like mayflies and caddisflies that feed on aquatic plants.
- Some fish species: Small fish that directly consume aquatic plants.
- Secondary Consumers (Primary Carnivores): These carnivores feed on the herbivores:
- Small Fish: Fish like minnows and sunfish that consume zooplankton and insect larvae.
- Larger Aquatic Insects: Insects that prey on other insects.
- Tertiary Consumers (Secondary Carnivores/Apex Predators): These are often the top predators in the lake:
- Larger Fish: Predators such as bass and pike that feed on smaller fish.
- Fish-eating Birds: Birds like herons and kingfishers that hunt fish.
- Decomposers: Bacteria and fungi break down dead organic matter, recycling nutrients within the lake ecosystem.
The dynamics of a lake food web are sensitive to environmental factors. For example, increased nutrient input from runoff (eutrophication) can lead to algal blooms, which can deplete oxygen levels and negatively impact the entire food web. This illustrates the interconnectedness of the different trophic levels.
Ultimate Conclusion
As the final curtain falls, we see that the blank food web is not just a static illustration; it’s a living, breathing entity. It adapts, it evolves, and it teaches us the delicate balance of nature. From the forest’s shadowed depths to the ocean’s sunlit surface, the connections are revealed. It’s a reminder that we are all interwoven, each thread crucial to the tapestry of life.
Embrace the power of the blank canvas, for within it lies the potential to comprehend the symphony of existence.