Food chain worksheet answers are your gateway to a fascinating journey into the heart of ecosystems! Prepare to be amazed as we explore the intricate relationships between living organisms, from the tiniest producers to the largest predators. This isn’t just about memorizing terms; it’s about understanding how energy flows, how ecosystems function, and how every creature plays a vital role.
We’ll dive deep into the fundamental components: producers like plants, consumers of various levels (primary, secondary, tertiary), and the crucial decomposers. You’ll discover how energy transfers through trophic levels, learn about the 10% rule, and even construct your own food chains and webs. We’ll also examine the impact of environmental changes, human activities, and the fascinating adaptations that make each organism unique.
Get ready to unlock the secrets of the natural world!
Defining the Food Chain
The food chain is a fundamental concept in ecology, illustrating the flow of energy and nutrients through an ecosystem. It demonstrates the relationships between organisms based on what they eat, connecting all living things in a complex web of interdependence. Understanding the food chain is crucial to appreciating how ecosystems function and how changes in one part of the chain can affect the whole system.
Basic Components of a Food Chain
The food chain comprises three main components: producers, consumers, and decomposers. Each component plays a vital role in the flow of energy and the cycling of nutrients within the ecosystem.* Producers: These are organisms that create their own food through photosynthesis or chemosynthesis. They form the base of the food chain.
Consumers
These organisms obtain their energy by consuming other organisms. Consumers are further classified into different levels based on what they eat: primary consumers (herbivores), secondary consumers (carnivores or omnivores that eat primary consumers), and tertiary consumers (carnivores that eat secondary consumers).
Decomposers
These organisms break down dead plants and animals, returning essential nutrients to the environment. They are crucial for nutrient cycling and ecosystem health.
Examples of Producers in Different Ecosystems
Producers vary depending on the ecosystem. Their ability to create food sustains all other life forms in the ecosystem. Here are examples in different environments:* Aquatic Ecosystems:
Phytoplankton
Microscopic, photosynthetic organisms that drift in the water. They are the primary producers in most aquatic environments. (Imagine a vast ocean, and visualize countless tiny, green algae, barely visible to the naked eye, absorbing sunlight and converting it into energy. This is phytoplankton at work.)
Seaweed
Large, multicellular algae, like kelp forests, provide habitat and food. (Picture the kelp forests swaying gently in the currents, creating a dense underwater forest where various marine animals find shelter and food.)
Terrestrial Ecosystems
Plants
Trees, shrubs, grasses, and other plants that utilize photosynthesis to produce their own food. (Consider a vast savanna, with tall grasses absorbing sunlight and providing sustenance for grazing animals.)
Mosses and Ferns
Found in various habitats, they also produce food through photosynthesis. (Envision a lush rainforest floor, where mosses and ferns thrive in the shade, creating a rich, diverse ecosystem.)
Energy Flow Through a Food Chain
Energy flows through a food chain in a unidirectional manner, from the producers to the consumers, and eventually to the decomposers. This flow is governed by the laws of thermodynamics, which dictate that energy is lost at each trophic level.
The 10% Rule: Only about 10% of the energy from one trophic level is transferred to the next. The remaining energy is used for life processes (metabolism, movement, etc.) or lost as heat.
For example, a primary consumer (herbivore) obtains energy by eating a producer (plant). The herbivore then becomes food for a secondary consumer (carnivore). As the energy moves up the food chain, the amount of energy available decreases at each level. This explains why there are typically fewer organisms at higher trophic levels. Consider a small pond ecosystem: sunlight provides energy for the algae (producer).
Small fish (primary consumers) eat the algae, and larger fish (secondary consumers) eat the small fish. A heron (tertiary consumer) may then eat the larger fish. At each step, a portion of the energy is lost.
Levels of Consumers
In the vibrant tapestry of a food chain, consumers play a crucial role in transferring energy. These organisms obtain their nourishment by consuming other living beings. The classification of consumers is based on their position in the food chain, indicating what they eat and how they acquire energy. Understanding these levels helps us grasp the intricate relationships within an ecosystem.
Types of Consumers
Consumers are broadly categorized into primary, secondary, and tertiary levels, each playing a unique role in energy flow.Primary consumers are the first level of consumers in a food chain. They are primarily herbivores, feeding directly on producers, such as plants. These organisms convert the energy stored in producers into a form they can use.Secondary consumers obtain their energy by feeding on primary consumers.
They can be carnivores or omnivores, depending on their diet. This level marks a shift in energy transfer, as the energy initially captured by producers moves up the food chain.Tertiary consumers are the top-level consumers in a food chain. They typically feed on secondary consumers and are often apex predators. These consumers play a vital role in regulating the populations of lower-level consumers.
Comparison of Herbivores, Carnivores, and Omnivores
The diet of consumers significantly influences their role in the ecosystem. The following table illustrates the differences between herbivores, carnivores, and omnivores.
Consumer Type | Diet | Examples | Role in the Ecosystem |
---|---|---|---|
Herbivores | Primarily plants (producers) | Deer, rabbits, caterpillars | Convert plant energy into a form usable by higher-level consumers. |
Carnivores | Other animals (consumers) | Wolves, lions, hawks | Control populations of herbivores and other carnivores. |
Omnivores | Both plants and animals | Bears, humans, raccoons | Exhibit flexibility in food sources, adapting to seasonal changes and food availability. |
Consumer Levels in a Forest Ecosystem
A forest ecosystem provides an excellent example to observe the various consumer levels in action. The following table showcases examples within a forest ecosystem.
Consumer Level | Examples | Description |
---|---|---|
Primary Consumers | Deer, Squirrels, Caterpillars | These animals directly consume plants, such as leaves, seeds, and fruits, converting plant energy into their own energy. |
Secondary Consumers | Foxes, Owls, Snakes | These animals prey on primary consumers, such as squirrels and rabbits. They obtain energy by consuming herbivores. |
Tertiary Consumers | Hawks, Wolves | Apex predators that consume secondary consumers. They regulate the populations of animals below them in the food chain. |
Energy Transfer and Trophic Levels
Energy flows through a food chain, driving all life processes. This transfer is not perfectly efficient; energy is lost at each step. Understanding trophic levels and the efficiency of energy transfer is crucial for comprehending ecosystem dynamics and the interconnectedness of organisms.
Trophic Levels and Energy Flow
Trophic levels represent the feeding positions in a food chain. Each level indicates the organism’s role in obtaining energy.
- Producers form the first trophic level, converting sunlight into energy through photosynthesis. Examples include plants and algae.
- Primary consumers, or herbivores, occupy the second level, feeding on producers. Examples include grasshoppers and rabbits.
- Secondary consumers, or carnivores, are the third level, consuming primary consumers. Examples include snakes and foxes.
- Tertiary consumers, also carnivores, are at the fourth level, preying on secondary consumers. Examples include eagles and sharks.
Energy flows from one trophic level to the next. Producers capture energy from the sun. Herbivores eat the producers, obtaining a portion of the energy. Carnivores eat the herbivores, and so on. However, not all energy is transferred; some is lost at each level.
The 10% Rule
The 10% rule describes the efficiency of energy transfer between trophic levels. This rule states that only about 10% of the energy from one trophic level is transferred to the next. The remaining 90% is lost.
- Energy loss occurs through various processes, including:
- Respiration: Organisms use energy for their metabolic activities, releasing it as heat.
- Waste: Undigested materials and waste products contain energy that is not transferred.
- Movement: Energy is expended during movement and other activities.
- This limited energy transfer has significant implications:
- Fewer organisms are supported at higher trophic levels because less energy is available.
- Food chains are typically shorter, with fewer trophic levels.
- The biomass (total mass of organisms) decreases at each higher trophic level.
The 10% rule is a general guideline, and the actual percentage can vary depending on the specific ecosystem and organisms involved.
The 10% rule: Only about 10% of the energy from one trophic level is transferred to the next.
Diagram of Energy Flow
The following describes an energy flow diagram for a simple food chain in a grassland ecosystem.
The diagram depicts a flow of energy through a grassland ecosystem. At the base of the food chain are the producers: green grasses. They are represented with a broad green shape at the bottom of the diagram, indicating a large energy input from the sun (represented by a yellow sun symbol above the grass). An arrow shows energy flowing from the sun to the grass.
Next, a primary consumer, a grasshopper, is depicted as a small green insect shape, consuming the grass. An arrow shows energy flowing from the grass to the grasshopper. A secondary consumer, a small brown bird (e.g., a sparrow), is then shown, eating the grasshopper. An arrow indicates energy transfer from the grasshopper to the bird. Finally, a tertiary consumer, a hawk, is illustrated at the top of the diagram.
The hawk, a large bird of prey, consumes the small bird. An arrow demonstrates the energy transfer from the small bird to the hawk. The size of each shape (grass, grasshopper, bird, hawk) progressively decreases, illustrating the decreasing amount of energy available at each successive trophic level. Beside each organism, there are values in parentheses, showing the relative amount of energy available (e.g., if the grass has 10,000 units of energy, the grasshopper might have 1,000, the bird 100, and the hawk 10, reflecting the 10% rule).
Each arrow also shows that a significant amount of energy is lost as heat (represented by small red “heat” symbols) at each transfer step, indicating the inefficiency of energy transfer.
Food Webs vs. Food Chains: Food Chain Worksheet Answers
The interconnectedness of life in any ecosystem is beautifully complex. While food chains offer a simplified view of energy transfer, food webs represent a more accurate and nuanced understanding of these relationships. This section delves into the distinctions between these two models, exploring their structure, stability, and how they reflect the intricate dynamics of nature.
Distinguishing Food Chains and Food Webs
Food chains and food webs, though both representing energy flow in an ecosystem, differ significantly in their complexity and representation. A food chain is a linear sequence, a single path showing the flow of energy from one organism to the next. A food web, in contrast, is a complex network of interconnected food chains, depicting the multiple feeding relationships within a community.
Food Chain | Food Web |
---|---|
Represents a single, linear pathway of energy transfer. | Represents multiple, interconnected pathways of energy transfer. |
Simplified model, showing a single predator-prey relationship. | Complex model, showing many predator-prey relationships and the feeding interactions of all organisms in an ecosystem. |
Easier to understand but less realistic. | More realistic but more complex. |
Vulnerable to disruptions; removing one organism can significantly impact the entire chain. | More resilient to disruptions; the removal of one organism is less likely to cause widespread ecosystem collapse because alternative food sources exist. |
Examples of Interconnected Food Chains
Food webs are constructed by connecting multiple food chains together. Let’s consider a simplified coastal ecosystem as an example.
- Food Chain 1: Phytoplankton (Producer) → Small Fish (Primary Consumer) → Larger Fish (Secondary Consumer) → Shark (Tertiary Consumer)
- Food Chain 2: Seaweed (Producer) → Sea Urchin (Primary Consumer) → Sea Otter (Secondary Consumer) → Shark (Tertiary Consumer)
- Food Chain 3: Phytoplankton (Producer) → Zooplankton (Primary Consumer) → Small Fish (Secondary Consumer) → Seabird (Tertiary Consumer)
- Food Chain 4: Seaweed (Producer) → Limpet (Primary Consumer) → Seabird (Secondary Consumer)
These individual food chains, when linked together, create a food web. For instance, the shark in Food Chain 1 can also be a predator in Food Chain 2, creating a connection between the two chains. Similarly, the small fish in Food Chain 1 can be prey for the seabird in Food Chain 3, establishing another connection. The sea otter in Food Chain 2 could also consume small fish in Food Chain 1.
Stability of Food Webs Compared to Food Chains
Food webs demonstrate greater stability compared to food chains because of their redundancy and interconnectedness. This means that if one organism is removed from a food web, the ecosystem is less likely to collapse compared to a food chain.The stability comes from several factors:
- Alternative Food Sources: Predators often have multiple prey options. If one prey species declines, the predator can switch to another.
- Energy Transfer Pathways: Energy can flow through multiple routes, ensuring that energy continues to be transferred even if one pathway is disrupted.
- Resilience to Disturbances: The complexity of food webs allows them to withstand environmental changes or species loss more effectively.
For example, consider the case of the Yellowstone National Park. The reintroduction of wolves led to a cascading effect throughout the food web. The wolves hunted elk, which had been overgrazing vegetation along riverbanks. With fewer elk, the vegetation recovered, which then benefited beavers. Beavers built dams, creating new habitats for fish, amphibians, and birds.
This illustrates how the complex interactions within a food web can result in significant changes and how the web’s resilience allowed the ecosystem to adapt and thrive.
Ecosystem Interactions
Ecosystems are dynamic systems, constantly shaped by interactions between living organisms and their environment. These interactions are crucial for the stability and function of food chains and webs. Understanding these interactions is essential for comprehending how ecosystems respond to change and how human activities impact their delicate balance. Environmental shifts, pollution, and the introduction of new species can trigger cascading effects, altering the flow of energy and the survival of organisms at various trophic levels.
Environmental Changes and Food Chains
Environmental changes can significantly alter food chains and webs, leading to both immediate and long-term consequences. Changes in climate, such as variations in temperature or precipitation, can disrupt the timing of biological events, such as plant flowering or insect emergence, which can impact the availability of food for consumers.
- Climate Change: Rising global temperatures can lead to habitat loss and shifts in species distributions. For example, the melting of Arctic sea ice directly impacts polar bears, which rely on the ice to hunt seals, their primary food source. A decrease in sea ice reduces hunting opportunities, leading to malnutrition and population decline.
- Natural Disasters: Events like wildfires, floods, and droughts can decimate populations of producers and consumers alike. A wildfire, for instance, can destroy vast areas of vegetation, removing the primary food source for herbivores and subsequently impacting the carnivores that prey on them. The sudden loss of habitat and food can cause mass migrations or even local extinctions.
- Habitat Destruction: Deforestation, urbanization, and agricultural expansion lead to habitat loss and fragmentation. This reduces the carrying capacity of an environment, limiting the resources available to support a diverse array of species. Reduced biodiversity can make ecosystems more vulnerable to further disturbances.
Pollution and Trophic Levels
Pollution introduces harmful substances into the environment, affecting organisms at all trophic levels. The impact of pollution can vary depending on the type of pollutant, its concentration, and the sensitivity of the organisms exposed to it.Here is a table showing how pollution affects organisms at different trophic levels:
Trophic Level | Pollutant | Example | Effect |
---|---|---|---|
Producers (Plants) | Acid Rain | Sulfur dioxide and nitrogen oxides from industrial emissions. | Damages leaf tissues, inhibits photosynthesis, and reduces plant growth. This reduces the base of the food chain. |
Primary Consumers (Herbivores) | Pesticides (e.g., DDT) | Pesticides sprayed on crops that are consumed by herbivores. | Bioaccumulation of toxins in the body, leading to reproductive problems, neurological damage, and potential death. For example, DDT accumulation thinned the eggshells of birds of prey, leading to population declines. |
Secondary Consumers (Carnivores) | Heavy Metals (e.g., Mercury) | Mercury released from industrial activities that contaminates water bodies and accumulates in fish. | Biomagnification: Higher concentrations of toxins in the bodies of predators that consume contaminated prey. This can cause neurological damage and reproductive failure. The Minamata disease outbreak in Japan is a stark example of mercury poisoning. |
Tertiary Consumers (Top Predators) | Plastic Waste | Plastic debris ingested by marine mammals and seabirds. | Physical harm (e.g., entanglement, choking) and the accumulation of toxic chemicals that leach from the plastic. This can lead to starvation and reduced reproductive success. |
Introduction of New Species and Food Webs
The introduction of a new species, whether intentional or accidental, can have profound and often unpredictable consequences for food webs. Invasive species can outcompete native organisms for resources, prey on native species, or alter habitats, leading to significant disruptions in ecosystem structure and function.
- Competition: The introduction of the zebra mussel ( Dreissena polymorpha) into the Great Lakes provides a case of strong competition. Zebra mussels are highly efficient filter feeders, outcompeting native mussels and other aquatic organisms for food. This has altered the food web, impacting phytoplankton populations, affecting the populations of other filter feeders and impacting the food available for fish species.
- Predation: The introduction of the brown tree snake ( Boiga irregularis) to Guam is a well-known example of predation. The snake, which has no natural predators on the island, has decimated native bird and reptile populations. This has led to cascading effects, including the decline of forest regeneration, as birds were important seed dispersers.
- Disease: Invasive species can introduce novel diseases that native species have not evolved defenses against. The chytrid fungus ( Batrachochytrium dendrobatidis) has caused widespread amphibian declines and extinctions globally. The fungus infects the skin of amphibians, disrupting their ability to absorb water and electrolytes.
Decomposers and the Cycle of Matter
Decomposers are the unsung heroes of any ecosystem, playing a vital role in the continuous recycling of nutrients. Without their tireless work, dead organisms and waste products would accumulate, locking up essential elements and hindering the flow of energy through the system. This section delves into the crucial role of decomposers, comparing their activities across diverse environments and highlighting the invaluable benefits they provide.
Find out further about the benefits of medway ma food pantry that can provide significant benefits.
The Role of Decomposers in Breaking Down Organic Matter
Decomposers, a diverse group including bacteria, fungi, and some animals, are the primary agents of decay. Their work involves breaking down complex organic molecules from dead organisms and waste into simpler substances. This process, known as decomposition, releases nutrients back into the environment, making them available for producers like plants. The efficiency and rate of decomposition are affected by several factors.
- Enzymatic Action: Decomposers secrete enzymes that break down organic matter. These enzymes act as biological catalysts, speeding up the chemical reactions involved in decomposition. For example, cellulase enzymes, produced by fungi and bacteria, break down cellulose, the main component of plant cell walls.
- Nutrient Release: As organic matter decomposes, essential nutrients like nitrogen, phosphorus, and potassium are released. These nutrients are then absorbed by plants, fueling their growth. This process ensures a continuous supply of nutrients within the ecosystem.
- Humus Formation: Decomposition also leads to the formation of humus, a stable, dark-colored organic matter. Humus improves soil structure, water retention, and nutrient availability, making it essential for plant growth.
Comparing the Process of Decomposition in Different Environments
The process of decomposition varies significantly depending on the environment. Factors such as temperature, moisture, oxygen availability, and the type of organic matter present all influence the rate and efficiency of decomposition.
- Compost Pile: In a compost pile, decomposition is typically rapid due to optimal conditions. A compost pile is often warm and moist, and it provides a rich source of organic matter. Aerobic decomposition (with oxygen) is the dominant process, leading to faster breakdown. The resulting compost is rich in nutrients and beneficial for plant growth. A compost pile is often manually managed, with regular turning to ensure oxygen availability.
The presence of diverse decomposers, including bacteria, fungi, and invertebrates like earthworms, contributes to the efficiency of the process.
- Ocean Floor: Decomposition on the ocean floor is generally slower than in a compost pile. The deep-sea environment is cold, dark, and often oxygen-poor. Anaerobic decomposition (without oxygen) becomes more prevalent, leading to slower breakdown rates. The types of decomposers present are also different, with specialized bacteria playing a crucial role. The process can be extremely slow, especially in areas with little oxygen, leading to the accumulation of organic matter over long periods.
The pressure at these depths also impacts the process.
- Forest Floor: In a forest floor environment, decomposition is often moderate. Temperature and moisture levels vary depending on the season and location. Leaf litter and other organic matter decompose through the action of fungi, bacteria, and invertebrates. The presence of oxygen allows for relatively efficient decomposition, but the rate can be slower than in a compost pile due to lower temperatures and less active management.
The specific types of trees and the climate of the forest also influence the decomposition rate.
Identifying the Benefits of Decomposition for an Ecosystem
Decomposition provides numerous benefits that are essential for the health and stability of any ecosystem. The processes that decomposers carry out have profound and far-reaching effects.
- Nutrient Recycling: Decomposition releases essential nutrients that would otherwise be locked up in dead organisms. This recycling of nutrients ensures a continuous supply for producers, supporting plant growth and overall ecosystem productivity. Without this, the ecosystem would rapidly deplete its resources.
- Waste Removal: Decomposers break down dead organisms and waste products, preventing the accumulation of harmful substances and creating a cleaner environment. This prevents the buildup of disease-causing agents and unpleasant odors.
- Soil Formation: Decomposition contributes to soil formation by producing humus. Humus improves soil structure, water retention, and nutrient availability, making it crucial for plant growth and supporting a healthy soil ecosystem.
- Energy Transfer: Decomposition facilitates the transfer of energy from dead organisms to decomposers and ultimately back into the food web. Decomposers themselves become a food source for other organisms, further cycling energy within the ecosystem.
Constructing Food Chains and Webs
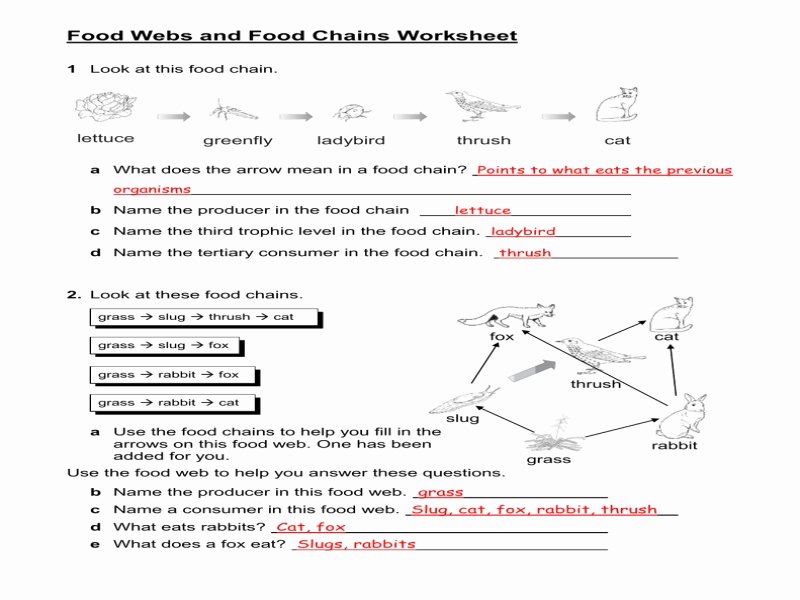
Understanding how energy flows through ecosystems is fundamental to grasping ecological relationships. Constructing food chains and webs allows us to visualize and analyze these complex interactions, revealing the interconnectedness of life and the impact of changes within an environment.
Constructing a Food Chain Procedure
The construction of a food chain follows a structured approach, starting with identifying the producers and culminating in the apex predator.
- Identify the Producers: Begin by pinpointing the autotrophs, such as plants or algae, which are the foundation of the food chain. These organisms create their own food through photosynthesis. For example, in a terrestrial ecosystem, the producers might be grasses or trees.
- Determine the Primary Consumers: Next, identify the herbivores, the organisms that consume the producers. These are the primary consumers. Examples include grasshoppers that eat grass or deer that eat leaves.
- Identify the Secondary Consumers: Proceed to the secondary consumers, which are carnivores or omnivores that eat the primary consumers. This could include a snake that eats a mouse or a fox that eats a rabbit.
- Locate the Tertiary Consumers (and beyond): Continue to identify higher-level consumers. Tertiary consumers are carnivores that eat secondary consumers, and so on. An example is a hawk that eats a snake.
- Represent the Energy Flow: Use arrows to indicate the direction of energy flow. Each arrow points from the organism being eaten to the organism that is eating it. The direction of the arrow always signifies “is eaten by”.
- Finalize the Chain: The food chain concludes with the apex predator, an organism at the top of the chain that is not preyed upon by other organisms within that specific food chain.
Food Web Template
A food web is a more complex representation of feeding relationships, showing interconnected food chains. Creating a template helps organize and visualize these relationships.
The food web template below provides a structured format for building a food web. It includes a list of organisms and a matrix to indicate feeding relationships. Note that organisms can occupy multiple positions within the web, acting as both prey and predator.
Organism | Producer | Primary Consumer | Secondary Consumer | Tertiary Consumer |
---|---|---|---|---|
Grass | Yes | |||
Rabbit | Yes | |||
Fox | Yes | |||
Hawk | Yes | Yes | ||
Grasshopper | Yes | |||
Snake | Yes |
Instructions for use:
- List the organisms involved in the ecosystem in the first column.
- For each organism, mark “Yes” in the appropriate columns to indicate its role in the food web. For example, a grasshopper is a primary consumer, so mark “Yes” in the “Primary Consumer” column.
- Arrows can then be drawn between the organisms based on the “Yes” marks, illustrating the energy flow.
Analyzing Food Webs for Energy Flow and Relationships
Analyzing a food web reveals the complex interconnections and energy flow within an ecosystem, highlighting the impact of changes within the web.
Analyzing a food web involves several key steps.
- Trace Energy Pathways: Follow the arrows to trace the flow of energy through the web. Starting from the producers, track how energy moves through various consumers. This reveals the feeding relationships and trophic levels of each organism.
- Identify Trophic Levels: Determine the trophic level of each organism. Producers are at the first trophic level, primary consumers at the second, secondary consumers at the third, and so on. This categorization helps understand the hierarchy of feeding relationships.
- Analyze the Impact of Disruptions: Consider the effects of removing or adding organisms. For example, if a primary consumer like a rabbit is removed, it could decrease the food available for secondary consumers and lead to an increase in the producer population.
- Evaluate Biodiversity and Stability: Assess the biodiversity within the food web. A diverse food web, with multiple pathways for energy flow, tends to be more stable because if one food source disappears, other options are available.
- Assess Biomagnification Potential: Observe the potential for biomagnification, where toxins accumulate in higher trophic levels. As predators consume multiple prey, they ingest the toxins present in their prey, leading to higher concentrations in the predators.
Adaptations in Food Chains
Organisms within a food chain exhibit a remarkable array of adaptations that enable them to thrive in their specific roles. These adaptations are the result of natural selection, favoring traits that enhance an organism’s ability to obtain resources, avoid predation, and reproduce successfully. The intricate interplay of these adaptations shapes the structure and dynamics of food chains, driving the evolution of diverse life forms.
Predator-Prey Adaptations
Predator-prey relationships are a fundamental component of food chains, and the evolutionary arms race between predators and their prey has led to a fascinating display of adaptations. These adaptations can involve physical characteristics, behavioral traits, and physiological mechanisms.
- Camouflage: Many organisms have evolved camouflage, allowing them to blend seamlessly with their environment. This adaptation provides a significant advantage for both predators and prey. For example, the Maluku giant gecko ( Gehyra marginata) exhibits cryptic coloration, blending in with tree bark to ambush insects. Similarly, prey animals like the praying mantis can mimic leaves, avoiding detection by predators.
- Speed and Agility: Speed and agility are crucial adaptations for both predators and prey. Predators use speed to chase and capture prey, while prey utilize speed to escape. The speed of a Maluku flying fox ( Pteropus chrysoproctus) allows it to forage efficiently for fruit. The swiftness of a monitor lizard ( Varanus salvadorii) helps it to hunt smaller animals.
- Sharp Claws and Teeth: Predators often possess sharp claws and teeth designed for capturing and killing prey. These adaptations are essential for efficiently dismembering and consuming food. For instance, the claws of a civet cat ( Paradoxurus hermaphroditus) enable it to climb trees to hunt for bird eggs and other small animals. The teeth of a crocodile ( Crocodylus porosus) are perfect for gripping and tearing flesh.
- Venom: Some predators, such as the Moluccan cobra ( Naja sputatrix), have evolved venom to subdue their prey. Venom can paralyze or kill prey, making it easier for the predator to consume it. This is a highly specialized adaptation.
- Defensive Mechanisms: Prey species have developed various defensive mechanisms to avoid being eaten.
- Warning Coloration: Some animals use bright colors to warn predators of their toxicity or unpleasant taste. This is a form of aposematism. The poison dart frog’s vibrant hues serve as a clear warning.
- Spines and Armor: Certain animals, like the sea urchin, are covered in spines or hard shells for protection against predators.
- Mimicry: Some harmless species mimic the appearance of dangerous ones to deter predators. The viceroy butterfly mimics the monarch butterfly, which is poisonous.
Influence of Adaptations on Food Chain Structure
Adaptations significantly influence the structure of food chains by determining which organisms can interact and how effectively they can do so. These interactions shape the flow of energy and matter within an ecosystem.
- Specialization: Adaptations often lead to specialization, where organisms evolve to exploit specific resources or prey. This specialization can create distinct trophic levels and narrow the food chain. A Maluku Cuscus ( Phalanger orientalis) specializes in eating leaves and fruits.
- Coevolution: Predator-prey relationships can drive coevolution, where the adaptations of one species influence the evolution of another. For example, the speed of a predator might drive the evolution of faster prey.
- Trophic Cascades: Adaptations can influence the strength of trophic cascades, where changes at one trophic level have cascading effects on other levels. The presence or absence of a top predator, due to its adaptations, can significantly impact the abundance of other species.
- Food Web Complexity: Adaptations contribute to the complexity of food webs. The ability of organisms to exploit different resources and interact in various ways expands the number of links within a food web.
Human Impact on Food Chains
Human activities profoundly alter the delicate balance of food chains across the globe. These impacts, often driven by resource exploitation and environmental degradation, can lead to cascading effects, threatening biodiversity and ecosystem stability. Understanding these influences is crucial for developing effective conservation strategies.
Deforestation’s Influence, Food chain worksheet answers
Deforestation, the clearing of forests for agriculture, logging, and urbanization, severely disrupts food chains. The removal of trees eliminates habitats, reducing the availability of food and shelter for primary consumers like herbivores. This, in turn, affects the predators that rely on them.
- Habitat Loss: The direct destruction of forest ecosystems leads to a decline in the populations of various species. This reduction in species richness diminishes the complexity of food webs.
- Reduced Primary Productivity: Trees are the primary producers in forest ecosystems. Their removal diminishes the rate of photosynthesis and reduces the amount of energy available to fuel the food chain.
- Soil Erosion: Deforestation contributes to soil erosion, which can pollute waterways and negatively impact aquatic food chains. Sedimentation can smother aquatic plants and organisms, further disrupting the ecosystem.
- Example: In the Amazon rainforest, deforestation for cattle ranching and soybean cultivation has led to significant declines in populations of jaguars and other apex predators, as well as a reduction in the biodiversity of prey species like monkeys and tapirs.
Overfishing’s Effects
Overfishing, the removal of fish from a body of water at a rate that exceeds the natural replenishment rate, poses a significant threat to aquatic food chains. It can lead to the collapse of fish populations and destabilize entire marine ecosystems.
- Population Decline: Overfishing directly reduces the populations of targeted fish species. This can cause the population of other species, which rely on the targeted species as a food source, to decline.
- Bycatch: The incidental capture of non-target species, known as bycatch, can also disrupt food chains. These species, including marine mammals, seabirds, and turtles, can be killed or injured in fishing gear.
- Trophic Cascades: The removal of top predators can trigger trophic cascades, where the effects of overfishing cascade down the food chain. For example, the decline of predatory fish can lead to an increase in the populations of their prey, such as smaller fish or invertebrates, which may then overgraze on primary producers like algae, causing the ecosystem to change.
- Example: The collapse of the cod fishery in the Northwest Atlantic in the late 20th century provides a stark example of the devastating impacts of overfishing. The decline of cod, a top predator, led to an increase in the populations of their prey, such as shrimp and crabs, and altered the structure of the entire ecosystem.
Pollution’s Role
Pollution, stemming from various sources such as industrial waste, agricultural runoff, and plastic debris, introduces harmful substances into ecosystems. These pollutants can bioaccumulate and biomagnify, posing significant risks to organisms at higher trophic levels.
- Bioaccumulation: Pollutants like heavy metals (e.g., mercury) and persistent organic pollutants (e.g., pesticides) accumulate in the tissues of organisms.
- Biomagnification: As these pollutants move up the food chain, their concentrations increase, with apex predators often accumulating the highest levels. This can lead to reproductive problems, immune system dysfunction, and even death.
- Habitat Degradation: Pollution can degrade habitats, making them unsuitable for many species. For instance, chemical spills can contaminate waterways, harming aquatic organisms.
- Example: The contamination of the Great Lakes with mercury from industrial sources has led to elevated mercury levels in fish, posing a health risk to humans and wildlife that consume them.
Climate Change’s Impact
Climate change, driven by the emission of greenhouse gases, is altering global temperatures, weather patterns, and ocean conditions, leading to significant impacts on food chains.
- Shifting Species Distributions: As temperatures rise, species are forced to migrate to find suitable habitats. This can disrupt established food chains as species interactions change.
- Changes in Phenology: Climate change can alter the timing of biological events, such as the emergence of insects or the flowering of plants. This can lead to mismatches between predators and their prey, disrupting food chains.
- Ocean Acidification: The absorption of carbon dioxide by the oceans is causing ocean acidification, which threatens marine organisms, particularly those with calcium carbonate shells or skeletons, like corals and shellfish. This can have cascading effects on marine food chains.
- Example: The warming of ocean waters has led to coral bleaching events, where corals expel the algae that live within them, leading to coral death. This affects the many species that rely on coral reefs for food and shelter.
Sustainable Practices for Mitigation
Adopting sustainable practices is crucial to mitigate the negative impacts of human activities on food chains. These practices aim to reduce environmental damage and promote the long-term health of ecosystems.
- Sustainable Forestry: Implementing sustainable forestry practices, such as selective logging and reforestation, can reduce deforestation and protect forest ecosystems.
- Sustainable Fisheries Management: Implementing regulations such as catch limits, fishing gear restrictions, and marine protected areas can help to prevent overfishing and protect marine ecosystems.
- Pollution Control: Reducing pollution through the implementation of regulations, waste management practices, and the development of cleaner technologies can protect ecosystems from harmful pollutants.
- Climate Change Mitigation: Reducing greenhouse gas emissions through the transition to renewable energy sources, energy efficiency measures, and carbon sequestration efforts is essential to address climate change and its impacts on food chains.
These practices include implementing catch limits, promoting sustainable forestry, controlling pollution, and reducing greenhouse gas emissions.
Restoring Disrupted Food Chains: Potential Solutions
Restoring disrupted food chains requires a multifaceted approach, including active restoration efforts, conservation initiatives, and community engagement.
- Habitat Restoration: Restoring degraded habitats, such as replanting forests or restoring wetlands, can provide food and shelter for wildlife and help to rebuild food chains.
- Species Reintroduction: Reintroducing native species to ecosystems where they have been extirpated can help to restore food chain dynamics.
- Invasive Species Control: Controlling invasive species, which can outcompete native species and disrupt food chains, is crucial for ecosystem restoration.
- Community Engagement: Engaging local communities in conservation efforts is essential for the long-term success of restoration projects. This includes educating communities about the importance of food chains, involving them in monitoring and management, and providing economic incentives for sustainable practices.
Habitat restoration, species reintroduction, and community engagement are vital components of restoring disrupted food chains.
Last Recap
In conclusion, our exploration of food chain worksheet answers has unveiled the dynamic and interconnected nature of life on Earth. We’ve seen how energy flows, how ecosystems thrive, and how even the smallest changes can have significant consequences. Armed with this knowledge, you’re now equipped to appreciate the delicate balance of nature and the importance of protecting our planet. Remember, understanding food chains is key to understanding the world around us, so keep exploring and keep learning!